.
The Appendages, Anatomy, and Relationships of Trilobites, by Percy Edward Raymond
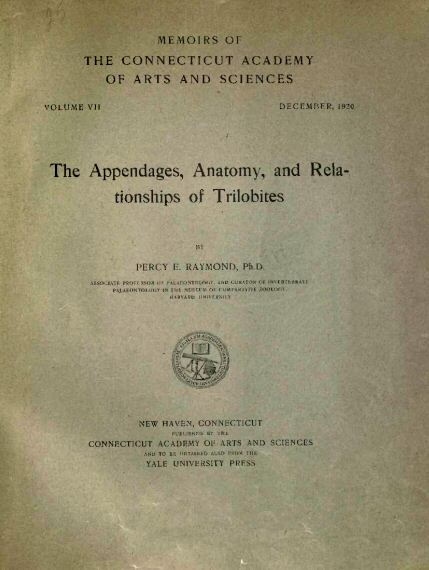
Transcriber's Note
Many figure captions contain size references (i.e., × 6) these should be taken as an approximate guideline as the displayed images most likely will not match the size of the originally printed versions due to a number of factors (monitor resolution, chosen display size in browser, etc.).
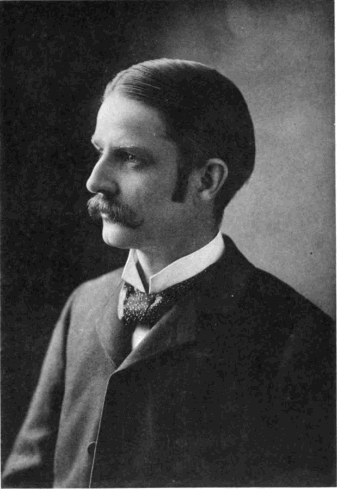
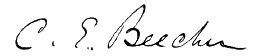
MEMOIRS OF
THE CONNECTICUT ACADEMY
OF ARTS AND SCIENCES
VOLUME VII DECEMBER, 1920
The Appendages, Anatomy, and Relationships
of Trilobites
BY
PERCY E. RAYMOND, Ph.D.
ASSOCIATE PROFESSOR OF PALAEONTOLOGY, AND CURATOR OF INVERTEBRATE
PALAEONTOLOGY IN THE MUSEUM OF COMPARATIVE ZOOLOGY,
HARVARD UNIVERSITY
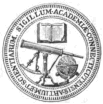
NEW HAVEN, CONNECTICUT
PUBLISHED BY THE
CONNECTICUT ACADEMY OF ARTS AND SCIENCES
AND TO BE OBTAINED ALSO FROM THE
YALE UNIVERSITY PRESS
THE TUTTLE, MOREHOUSE & TAYLOR COMPANY
TO THE MEMORY OF
CHARLES EMERSON BEECHER
SKILLFUL WITH HAND, BRAIN, AND PEN; REVEALER OF THE MYSTERIES OF TRILOBITES;
THIS MEMOIR IS DEDICATED
FOREWORD.
By CHARLES SCHUCHERT.
Trilobites are among the most interesting of invertebrate fossils and have long attracted the attention of amateur collectors and men of science. These "three-lobed minerals" have been mentioned or described in books at least since 1698 and now several thousand species are known to palæontologists. To this group of students they are the most characteristic animals of the seas of Palæozoic time, and even though they are usually preserved as dismembered parts, thousands upon thousands of "whole ones" are stored in the museums of the world. By "whole ones" perfect individuals are not meant, for before they became fossils the wear and tear of their time and the process of decomposition had taken away all the softer parts and even most of the harder exterior covering. What is usually preserved and revealed to us when the trilobites weather out of the embrace of their entombing rocks is the test, the hard shell of the upper or dorsal side. From time to time fragments of the under or limb-bearing side had been discovered, first by Elkanah Billings, but before 1876 there was no known place to which one could go to dig out of the ground trilobites retaining the parts of the ventral side.
Students of trilobites have always wanted specimens to be delivered to them weathered out of the rock by nature and revealing the ventral anatomy without further work than the collecting, but the wish has never been fulfilled. In the Utica black shales, near Rome, New York, there was finally discovered in 1892 a layer less than ten millimeters thick, bearing hundreds of Triarthrus becki with most of the ventral anatomy intact. The collector's first inkling that such were present in the Utica formation came to him in a chance find in 1884, and for eight years he sought off and on for the stratum whence this specimen came. His long search was finally rewarded by the discovery of the bed, and lo! here were to be had, in golden color, prostrate specimens with the breathing and crawling legs and the long and beautifully curved feeling organs all replaced by iron pyrites. Fool's gold in this case helped to make a palæontologic paradise. The bed contained not only such specimens of Triarthrus becki, but also, though more rarely, of Cryptolithus tessellatus and exceptionally of Acidaspis trentonensis. This important discovery, which has figured so largely in unraveling the evolution of the Crustacea and even has a bearing on that of most of the Arthropoda, was made by Mr. W. S. Valiant, then curator of the Museum of Rutgers College.
There were, however, great material difficulties to overcome before the specimens revealed themselves with all of their information exposed for study. No surgeon was needed, but a worker knowing the great scientific value of what was hidden, and with endless patience and marked skill in preparation of fossils. Much could be revealed with the hammer, because specimens were fairly abundant. A chance fracture at times showed considerable portions, often both antennæ entire, and more rarely the limbs protruding beyond the test, but the entire detail of any one limb or the variation between the limbs of the head, thorax, and tail was the problem to be solved. No man ever loved a knotty problem more than Charles E. Beecher. Any new puzzle tempted him, and this one of Triarthrus becki interested him most of all and kept him busy for years. From the summer of 1893. when he quarried out two tons of the pay stratum at Rome, until his death in 1904, his [6] time was devoted in the main to its solution by preparing these trilobites and learning their anatomical significance.
The specimens of Triarthrus becki from Rome are pseudomorphs composed of iron pyrites, as has been said, and are buried in a gray-black carbonaceous shale. A little rubbing of the specimens soon makes of them bronze images of the former trilobite and while under preparation they are therefore easily seen. However, as the average individual is under an inch in length and as all the limbs other than the antennæ are double or biramous, one lying over the other, and the outer one fringed with a filamentous beard, the parts to be revealed by the preparator are so small and delicate that the final touch often obliterates them. These inherent difficulties in the material were finally overcome by endless trials on several thousand specimens, each one of which revealed something of the ventral anatomy. Finally some 500 specimens worthy of detailed preparation were left, and on about 50 of these Beecher's descriptions of Triarthrus and Cryptolithus were based.
The black shale in which the specimens are buried is softer than the pseudomorphous trilobites, a condition that is of the greatest value in preparation. With chisel and mallet the trilobites are sought in the slabs of shale and then with sharp chisels of the dental type they are revealed in the rough. At first Beecher sought to clean them further by chemical methods, and together with his friends, the chemist Horace L. Wells, and the petrologist Louis V. Pirsson, several solutions were tried, but in all cases the fossils were so much decomposed as to make them useless in study. Therefore Beecher had to depend wholly oh abrasives applied to the specimens with pieces of rubber. Much of this delicate work was done on a dental lathe, but in the final cleaning most of it was done with patient work by hand. Rubber has the great advantage of being tough and yet much softer than either specimen or shale. As the shale is softer than the iron pyrites, the abrasives (carborundum, emery, or pumice) took away the matrix more quickly than the trilobite itself. When a part was fully developed, the rubbers were cut to smaller and smaller dimensions and the abrading reduced to minute areas. So the work went on and on, helped along from time to time by the dental chisels. Finally Beecher became so expert with these fossils that after one side was developed he would embed the specimen in Canada balsam and fix it on a glass slide, thus enabling him to cut down from the opposite side. This was done especially with Cryptolithus because of the great scarcity of material preserving the limbs, and two of these revealed both sides of the individuals, though they were then hardly thicker than writing paper.
Then came illustrations, which at first were camera-lucida drawings in pencil smoothed out with pen and ink. "In some quarters," however, it has been said, "his methods unknown, their results were not accepted; they were regarded as startling, as iconoclastic, and even unreliable." He therefore decided to rework his material and to illustrate his publications with enlarged photographs. The specimens were black, there was little relief between fossil and matrix, and the ammonium chloride process of coating them white and photographing under artificial light was unsuitable. Nevertheless, after many trials, he finally succeeded in making fine enlarged photographs of the trilobites immersed in liquid Canada balsam, with a contact cover of glass through which the picture was taken, the camera standing vertically over the horizontal specimen. Beecher had completed this work in 1903 and in the winter of 1903-1904 was making the drawings, nearly all of which are here reproduced. On Sunday morning, February 14, 1904, as he was working at home on a large wash drawing of Cryptolithus, death came to him suddenly, leaving the trilobite problem but partially solved.
When the writer, in the autumn of 1904, succeeded Professor Beecher in the chair of Palæontology at Yale, he expected to find considerable manuscript relating to the ventral anatomy of the trilobites, but there was only one page. It was Beecher's method first to prepare and thoroughly study the material in hand, then to make the necessary illustrations, and between times to read what others had written. There was no written output until everything had been investigated and read, certain passages being marked for later reference. Then when all was assimilated, he would write the headings of topics as they came to him, later cutting them apart and arranging them in a logical sequence. When the writer visited him in his home in January 1904, he was primed for his final trilobite memoir, but the writing of it had not been begun.
The writer has never made the trilobites his special subjects for study as he has the brachiopods, and therefore felt that he should not try to bring to light merely the material things that Beecher had so well wrought out. It seemed at first an impossible task to find the specialist and friend to do Beecher justice, but as the years have passed, one of Beecher's students, always especially interested in trilobites, has grown into a full appreciation of their structures and significance, and to him has fallen the continuation of his master's work. If in the following pages he departs here and there from the accepted interpretation and the results of others, it is because his scientific training, in desiring to see with his own eyes the structures as they are, has led him to accept only those interpretations that are based on tangible evidence as he understands such. Furthermore, in seeking the relationship of the trilobites to the rest of the Arthropoda, his wide study of material and literature, checked up by the ontogeny of fossil and recent forms, has led him in places from the beaten path of supposedly ascertained phylogenies. His results, however, have been won through a detailed study of the interrelations of the Arthropoda, starting from the fact that the Trilobita are chronogenetically the oldest and most primitive. The trilobites are held by him to be the most simple, generalized, ancient Crustacea known, and the progenitors, directly and indirectly, of all Arthropoda.
It is now twenty-six years since Professor Beecher began his publications on the class Trilobita, and in commemoration of him and his work, Professor Percy E. Raymond of Harvard University presents this memoir, to bring to fruition the studies and teachings of his honored guide. It has been with Professor Raymond a labor of love, and it is for the writer of this foreword a long-desired memorial to the man to whose position in the Museum and University he had the privilege of succeeding.
Yale University, New Haven, Connecticut.
PREFACE.
The primary object of this memoir is, as has been stated by Professor Schuchert, to. rescue from oblivion the results of the last few years of Professor Beecher's investigations on the ventral anatomy of trilobites. Since he left his data in the form of drawings and photographs, without even rough notes, it became necessary, in order to write a text to accompany the plates, to restudy the entire subject. Under these circumstances, it seemed best to include all that is known about the appendages of trilobites, thus bringing together a summary of present information on the subject.
The growth of the memoir to its present size has been a gradual one. As first completed in 1917, it contained an account of the appendages only. Thoughts upon the probable use of the appendages led to the discussion of possible habits, and that in turn to a consideration of all that is known or could be inferred of the structure and anatomy of the trilobite. Then followed an inquiry into the relationships to other Arthropoda, which ultimately upset firmly established preconceptions of the isolated position of the group, and led to a modification of Bernard's view of its ancestry.
During the progress of the work, I have had the opportunity of examining most of the known specimens retaining appendages. From the Marsh collection in the Yale University Museum were selected the forty-six specimens showing best the appendages of Triarthrus, Cryptolithus, and Acidaspis. Dr. Charles D. Walcott very kindly returned to the Museum of Comparative Zoology the slices of Ceraurus, Calymene, and Isotelus which were the basis of his paper of 1881, and which had been loaned him for further study. He loaned also eight of the more important specimens of Neolenus serratus, and two of Triarthrus becki. At the United States National Museum I saw the specimens of Isotelus described by Mickleborough and the isolated limbs of Calymene from near Cincinnati. The Isotelus at Ottawa I had already studied with some care while an officer of the Geological Survey of Canada.
This memoir consists, as shown in the table of contents, of four parts. The appendages of Neolenus, Isotelus, Ptychoparia, Kootenia, Ceraurus, Calymene, and Acidaspis are discussed, as fully as circumstances warrant, in the first part, and new restorations of the ventral surfaces of Neolenus, Isotelus, Triarthrus, Ceraurus and Cryptolithus are included It is not supposed that these restorations will be of permanent value in all of their detail, but they are put forward as the best approximations to the real structure that the writer is able to present from the materials so far discovered. I am greatly indebted to Doctor Elvira Wood for the care and skill with which she has worked up these restorations from my rather sketchy suggestions. She has put into them not only a great amount of patient work, but also the results of considerable study of the specimens.
Part II is a discussion of the internal anatomy of the trilobite and a brief statement of some of the possible habits and methods of life of these animals. Part III, which begins with a survey of the relationships of the trilobites to other Arthropoda, is largely taken up with an attempt to demonstrate the primitive characteristics of the former, and their probable ancestral position. The form of the ancestor of the trilobite is deduced from a study of the morphology, ontogeny, and phylogeny of the group, and evidence adduced to indicate that it was a depressed, flattened, free-swimming animal of few segments.
In Part IV are included somewhat detailed descriptions of a few of the best specimens of Triarthrus and Cryptolithus. Professor Beecher, while an observer of the minutest details, believed in publishing only the broader, more general results of his investigations. This method made his papers brief, readable, and striking, but it also resulted in leaving in some minds a certain amount of doubt about the correctness of the observations. In a matter so important as this, it has seemed that palæontologists are entitled to the fullest possible knowledge of the specimens on which the conclusions are based. The last part is, therefore, a record of the data for the restorations of Triarthrus and Cryptolithus.
The illustrations in the plates were nearly all made by or under the supervision of Professor Beecher, as were also text figures 45 and 46.
In conclusion, I wish to express my thanks to Mrs. Charles E. Beecher for the use of drawings which were the personal property of Professor Beecher; to Doctor Charles D. Walcott for photographs of the limbs of Calymene, and for his kindness in sending me the slices of trilobites from Trenton Falls and specimens of Neolenus and Triarthrus; to Doctor R. V. Chamberlin for suggestions and criticisms in regard to the relationship of trilobites to Insecta, Arachnida, Chilopoda, and Diplopoda; to Mr. Samuel Henshaw, Director of the Museum of Comparative Zoology, for permission to use the time which has been devoted to this work; and to Miss Clara M. Le Vene, for assistance in the preparation of the manuscript. My greatest debt is to Professor Charles Schuchert, to whom the work owed its inception, who has assisted in many ways during its prosecution, and who read the manuscript, and arranged for its publication. To him I can only express my warmest thanks for the favors which I have received and for the efforts which he has put forth to make this a worthy memorial to our friend and my teacher, Professor Charles Emerson Beecher.
Harvard University, Cambridge, Mass.
November, 1919.
TABLE OF CONTENTS.
LIST OF ILLUSTRATIONS.
1 | Triarthrus becki Green. Diagram of limb to show nomenclature employed | 20 |
2 | Neolenus serratus (Rominger). Two thoracic appendages | 24 |
3 | The same. An exopodite | 26 |
4 | The same. A so-called "epipodite" | 26 |
5 | The same. The so-called "exites" | 29 |
6 | The same. A cephalic limb | 29 |
7 | The same. Restoration of a transverse section | 30 |
8 | The same. Restoration of the ventral surface | 31 |
9 | Isotelus. Restoration of the ventral surface | 38 |
10 | Triarthrus becki Green. Restoration of the ventral surface | 41 |
11 | The same. Median appendage | 44 |
12 | Ceraurus pleurexanthemus Green. Slice showing an exopodite | 49 |
13 | Calymene senaria Conrad. Slice showing cephalic coxopodites | 53 |
14 | The same. Another similar slice | 53 |
15 | The same. Slice showing method of articulation of the appendages | 53 |
16 | The same. Restoration of the ventral surface | 55 |
17 | Ceraurus pleurexanthemus Green. Slice showing the method of articulation of the appendages | 58 |
18 | The same. Slice showing an exopodite above an endopodite | 58 |
19 | The same. Restoration of a transverse section | 60 |
20 | Cryptolithus tessellatus Green. Restoration of the ventral surface | 63 |
21 | Ceraurus pleurexanthemus Green. Slice showing the abdominal sheath | 79 |
22 | The same. Slice showing the large alimentary canal | 79 |
23 | Calymene senaria Green. Slice showing the large alimentary canal | 79[15] |
24 | Ceraurus pleurexanthemus Green. Restoration of a longitudinal section | 81 |
25 | Cryptolithus tessellatus Green. Cheek showing the genal cæca | 84 |
26 | Illænus. Volborth's figure of the heart | 85 |
27 | Heart of Apus | 85 |
28 | Isotelus gigas Dekay. The Panderian organs | 91 |
29 | Ceraurus pleurexanthemus Green. Restoration, showing heart, alimentary canal, and extensor muscles | 93 |
30 | The same. Longitudinal section of cephalon | 95 |
31 | Nileus armadillo Dalman. Moberg's figure of the muscle-scars | 95 |
32 | Marrella splendens Walcott. Restoration of the ventral surface | 116 |
33 | Triarthrus becki Green. Appendage of the anterior part of the thorax | 126 |
34 | Apus. Appendage from the anterior part of the trunk | 127 |
35 | Weymouthia nobilis (Ford) | 138 |
36 | Naraoia compacta Walcott | 145 |
37 | Pagetia clytia Walcott | 145 |
38 | Asaphiscus wheeleri Meek | 145 |
39 | Pædeumias robsonensis Burling | 145 |
40 | Robergia sp. | 145 |
41 | Diagram showing possible lines of descent of the Arthropoda | 150 |
42 | Triarthrus becki Green. Thoracic appendages | 155 |
43 | The same. Pygidial appendages | 157 |
44 | The same. Pygidial appendages | 158 |
45 | Cryptolithus tessellatus Green. Drawing of the best single specimen | 159 |
46 | The same. Part of the thorax and pygidium, with appendages | 162 |
Frontispiece. Charles Emerson Beecher, 1896.
Plates 1-5. Photographs of Triarthrus becki, made by C. E. Beecher.
Plate 6. Photographs of Triarthrus becki (figs. 1-3), Acidaspis trentonensis (fig. 6), and Cryptolithus tessellatus (fig. 7), made by C. E. Beecher. Photographs of the endopodites of a probable species of Calymene (figs. 4, 5)
Plates 7-8. Photographs of Cryptolithus tessellatus, made by C. E. Beecher.
Plate 9. Drawings of Cryptolithus tessellatus, made by C. E. Beecher or under his direction.
Plate 10. Photographs of Isotelus latus and I. maximus, made by C. E. Beecher.
Plate 11. Drawing of a restoration of Ceraurus pleurexanthemus, made by Elvira Wood.
HISTORICAL REVIEW.
The beginning of the search for the limbs of trilobites was coeval with the beginning of scientific study of the group, knowledge of the appendages being essential to the proper systematic allocation of the animals.
The early search was so barren of results that negative evidence came to be accepted as of positive value, and it was for many years generally believed that such organs as may have been present beneath the dorsal test were so soft as to be incapable of preservation. This view is best expressed by Burmeister (1846, p. 43):
There is good proof that the feet of trilobites must have been soft membranous organs, for the absence of the slightest remains of these organs in the numerous specimens observed is of itself evidence of the fact, and it can indeed scarcely be supposed that hard horny extremities should be affixed to a soft membranous abdominal surface; since they would not have possessed that firm basis, which all solid organs of locomotion require, in order that they may be properly available.
Very well reasoned, and were it not for the discovery of new material in American localities, Burmeister's views would probably never have been proved incorrect. One can not escape the suspicion that some of the accepted hypotheses of today, founded on similar "proof," may yield in time to the weight of bits of positive evidence.
The history of the study of appendages of trilobites may be divided into two periods. The first, in which there was a general belief that the appendages were soft organs, but during which numerous "finds" of limbs were reported, extended from the time of Linné to the year (1876) in which Walcott demonstrated the fact that the animals possessed jointed ambulatory and breathing organs.
The second, much more fruitful period, began with Walcott's publication of 1881, descriptive of the appendages of Ceraurus and Calymene, and for the purposes of this memoir, closes with his great contribution on the anatomy of Neolenus (1918). Beecher's brilliant productions came in the middle of the second period.
In the first period, there were at least two authentic discoveries of appendages, those of Eichwald (1825) and Billings (1870), but since neither of these men convinced his confreres of the value of his finds, the work of neither can be considered as having marked an especial epoch in the history.
As all the authentic finds will be treated in detail on later pages, only a brief résumé of the first period will be given here. This has already been done by Burmeister (1843, 1846) and Barrande (1852, 1872), whose works have been my primary sources of information, but I have looked up the original papers, copies of nearly all of which are to be seen in the libraries in Cambridge and Boston. Brig.-Gen. A. W. Vogdes, U. S. A. (retired), has very kindly placed at my disposal a number of references and notes.
Linné (1759) was the first to report the discovery of appendages of trilobites. Törnquist (1896) has pressed for a recognition of the contribution of the great Swedish naturalist to this problem, but Beecher (1896 B) doubted the validity of the find. Linné figured a specimen of Parabolina spinulosa (Wahlenberg), with what he interpreted as a pair of antennæ attached. He states (translation quoted from Törnquist): "Most remarkable in this specimen are the antennæ in the front, which I never saw in any other sample, and which clearly prove this fossil to belong to the insects." Beecher has shown as conclusively as can be shown without access to the original specimen that the supposed antennæ were really only portions of the thickened anterior border, the appearance being due to imperfect preservation. Brünnich as early as 1781 called attention to the imperfection of this specimen, [18] and it is also referred to by Wahlenberg (1821, p. 39), Brongniart (1822, p. 42), Dalman (1828, p. 73), and Angelin (1854, p. 46).
Audouin (1821) seems to have been the first naturalist with sufficient knowledge of the Arthropoda to be competent to undertake the study of the trilobites. He concluded that the absence of ventral appendages was probably a necessary consequence of the skeletal conformation, and thought if any were discovered, they would prove to be of a branchial nature.
Wahlenberg (1821) in the same year expressed his belief that the trilobites were nearly allied to Limulus and in particular tried to show that the trilobites could have had masticatory appendages attached about the mouth as in that modern "insect" (p. 20). Wahlenberg was also the first to describe an hypostoma of a trilobite (p. 37, pl. 1, fig. 6), but did not understand the nature of his specimen, which he described as a distinct species.
Brongniart (1822, p. 40) devoted five pages of his monograph to a discussion of the affinities of trilobites, concluding that it was very probable that the animals lacked antennæ and feet, unless it might be that they had short soft feet which would allow them to creep about and fix themselves to other bodies.
Schlotheim (1823) thought that the spines on Agnostus pisiformis were segmented and compared them with the antennæ of Acarus.
Stokes (1823) was the first who, with understanding, published an illustration of the ventral side of a trilobite, having figured the hypostoma of an Isotelus. He was followed in the next year (1824) by Dekay, who also figured the hypostoma of an Isotelus, and added some observations on the structure of trilobites. The researches of Barrande, Novak, Broegger, Lindstroem, and others have dealt so fully with the hypostoma that further references to that organ need not be included here.
Dalman (1826, 1828) reviewed the opinions of his predecessors, and thought it not impossible that organs of mastication may have been present under the head shield of the trilobite as in Limulus (1828, p. 18). In this he of course followed Wahlenberg.
Goldfuss (1828) figured sections of Dalmanites hausmanni, Phacops macrophthalma, and Calymene tristani, which remind one of some of Doctor Walcott's translucent slices. So far as one can judge from the illustrations, it is probable that what he took for limbs were really fragments of other trilobites. Such is certainly the case in his figures 9 and 10, where a number of more or less broken thoracic segments are present. The section of Encrinurus punctatus shown in figure 7 may possibly exhibit the position and folds of the ventral membrane beneath the axial lobe, and also, perhaps, the appendages. His figures 4, 5 and 8 show the hypostoma in section.
Pander (1830) described the hypostoma in greater detail than had been done by previous authors, but otherwise added nothing to the subject.
Sternberg (1830) thought he had individuals showing appendages, but judging from his poor figures, he was deceived by fragmentary specimens.
Green (1839 A, B, C) described specimens of Phacops from Berkeley Springs, West Virginia, which had the hypostoma in position, and appear to have had a tubular opening under the axial lobe. While appendages were not actually present, these specimens suggested fairly correct ideas about the swimming and breathing organs of trilobites. They were similar to the ones which Castelnau obtained, and all were perhaps from the same locality.
It is not worth while to do more than enumerate the other authors of this period: Hisinger 1837, Emmrich 1839, Milne-Edwards 1841, for they all shared the same views, and added nothing to what was already known.
Castelnau (1843) described and figured a Phacops said to come from Cacapon Springs, West Virginia, which he thought possessed remains of appendages. There is nothing in the description or figures to indicate exactly what was present, but it is very unlikely that any limbs were preserved. The broad thin "appendage" figured may have been a fragment of a thoracic segment. This specimen was evidently described by Castelnau before 1843, as is inferred from a reference in the Neues Jahrbuch, 1843, P. 504, but I have not seen the earlier publication.
Burmeister (1843-1846), in his "Organization of the Trilobites," reviewed in extenso the history of the search for appendages, and concluded that they must have been so soft as to preclude the possibility of their being preserved as fossils. "Their very absence in fossils most distinctly proves their former real structure" (p. 10). In figures 7 and 8 on plate 6 he gave a restoration of the ventral surface of an Asaphus, the first restoration of the ventral anatomy to be attempted. Since he chose modern branchiopods as his model, he did not go so far wrong as he might have done. Still, there is little in the figure that would now be accepted as correct. The following quotation will serve to give the opinion of this zoologist, who from his knowledge of the Crustacea, was the most competent of the men of his time to undertake a restoration of the appendages of the trilobites:
… in giving a certain form to the feet in the restored figure, I have done so rather intending to indicate what they might have resembled, than with any idea of assuming their actual form. I merely assert that these organs were soft, membranous, and fringed, adapted for locomotion in water, placed on the abdominal portion of the body, and extending sidewise beneath the lateral lobes of the rings, as shown in the ideal transverse section. These feet were also indented, and thus divided into several lobes at the open lower side, and each separate lobe was furnished at the margin with small bristles serving as fins. The last and external lobe was probably longer, smaller, and more movable, and reached to the termination of the projecting shell lobe, bearing a bladder-shaped gill on the inner side (1846, p. 45).
McCoy (1846) observed in several trilobites a pair of pores situated in the dorsal furrows near the anterior end of the glabella. He showed that the pits occupy precisely the position of the antennæ of insects and suggested that they indicated the former presence of antennæ in these trilobites (chiefly Anipyx and "Trinucleus"). The evidence from Cryptolithus, set forth on a later page, indicates the correctness of McCoy's view.
Richter (1848, p. 20, pl. 2, fig. 32) described and figured what he took to be a phyllopod-like appendage found in a section through a Phacops. Without the specimen it is impossible to say just what the structure really was. The outline figure is so obviously modeled on an appendage of Apus that one is inclined to think it somewhat diagrammatic. In calling attention to this neglected "find," Clarke (1888, p. 254, fig.) interprets the appendage as similar to the spiral branchiæ of Calymene senaria, and adds that he himself has seen evidence of spiral branchiæ in the American Phacops rana.
Beyrich (1846) described a cast of the intestine of "Trinucleus," and Barrande (1852) further elaborated on this discovery.
Corda (1847) made a number of claims for appendages, but all were shown by Barrande (1852) to be erroneous.
Barrande (1852, 1872) gave a somewhat incomplete summary of the various attempts to describe the appendages of trilobites, concluding that none showed any evidence of other than soft appendages, until Billings' discovery of 1870.
Volborth (1863) described a long chambered tubular organ in Illænus which he believed to represent a cast of the heart of a trilobite, but which has since been likened by writers to the intestinal tract in "Trinucleus."
PART I.
THE APPENDAGES OF TRILOBITES.
The terminology employed in the succeeding pages is essentially the same as that used by Beecher, with two new terms added. Beecher assigned to the various segments of the limbs the names suggested by Huxley, but sometimes used the name protopodite instead of coxopodite for the proximal one. It is obvious that he did not use protopodite in the correct sense, as indicating a segment formed by the fusion of the coxopodite and basipodite. The usage employed here is shown in figure 1.
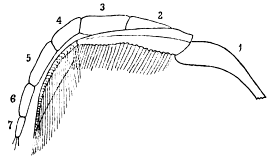
Fig. 1.—Triarthrus becki Green. Diagram of one of the limbs of the thorax, viewed from above, with the endopodite in advance of the exopodite. 1, coxopodite, the inner extension being the endobase (gnathobase on cephalon); 2, basipodite, springing from the coxopodite, and supporting the exopodite, which also rests upon the coxopodite; 3, ischiopodite; 4, meropodite; 5, carpopodite; 6, propodite; 7, dactylopodite, with terminal spines.
The investigation of Ceraurus showed that the appendages were supported by processes extending downward from the dorsal test, and on comparison with other trilobites it appeared that the same was true in Calymene, Cryptolithus, Neolenus, and other genera. Thin sections showed that these processes were formed by invagination of the test beneath the dorsal and glabellar furrows. While these processes are entirely homologous with the entopophyses of Limulus, I have chosen to apply the name appendifer to them in the trilobites.
The only other new term employed is the substitution of endobase for gnathobase in speaking of the inner prolongation of a coxopodite of the trunk region. The term gnathobase implies a function which can not in all cases be proved.
The individual portions of which the limbs are made up are called segments, and the articulations between them, joints. Such a procedure is unusual, but promotes clearness.
The first mention of Neolenus with appendages preserved was in Doctor Walcott's paper of 1911, in which two figures were given to show the form of the exopodites in comparison with the branchiæ of the eurypterid-like Sidneyia. In 1912, two more figures were presented, showing the antennules, exopodites, and cerci. The specimens were found in the Burgess shale (Middle Cambrian) near Field, in British Columbia. This shale is exceedingly fine-grained, and has yielded a very large fauna of beautifully preserved fossils, either unknown or extraordinarily rare elsewhere. It was stated in this paper (1912 A) that trilobites, with the exception of Agnostus and Microdiscus, were not abundant in the shale.
In discussing the origin of the tracks known as Protichnites, Walcott presented four figures of Neolenus with appendages, and described the three claw-like spines at the tip of each endopodite.
Three new figures of the appendages were also contributed to the second edition of the Eastman-Zittel "Text-book of Paleontology" (1913, p. 701). Later (1916, pl. 9) there was published a photograph of a wonderful slab, bearing on its surface numerous Middle Cambrian Crustacea. Several of the specimens of Neolenus showed appendages.
Finally, in 1918, appeared the "Appendages of Trilobites," in which the limbs of Neolenus were fully described and figured (p. 126), and a restoration presented. Organs previously unknown in trilobites, epipodites and exites, attached to the coxopodites, were found.
Illustrated: Walcott, Smithson. Misc. Coll., vol. 57, 1911, p. 20, pl. 6, figs. 1, 2 (exopodites of thorax and cephalon);—Ibid., vol. 57, 1912, p. 191, pl. 24, figs., 1, la (antennules, caudal rami, and endopodites of thorax);—Ibid., vol. 57, 1912, p. 277, pl. 45, figs. 1-4 (antennules, endopodites of cephalon and thorax, caudal rami);—Text-book of Paleontology, edited by C. R. Eastman, 2d ed., vol. 1. 1913, p. 701, fig. 1343 (exopodites), p. 716, fig. 1376 (abdominal appendages), fig. 1377 (appendages of thorax and pygidium);—Ann. Rept. Smithson. Inst. for 1915, 1916, pl. 9;—Smithson. Misc. Coll., vol. 67, 1918, pp. 126-131 et al., pl. 14, fig. 1; pls. 15-20; pl. 21, fig. 6; pls. 22, 23; pl. 31 (restoration); pl. 34, fig. 3 (restored section); pl. 35, fig. 4; pl. 36, fig. 3 (hypostoma).
The following description of the appendages of Neolenus is summarized from Walcott's paper of 1918, and from a study of the eight specimens mentioned below.
The antennules are long, slender, and flexible, and lack the formal double curvature so characteristic of those of Triarthrus. There are short fine spines on the distal rims of the segments of the proximal half of each, thus giving great sensitiveness to these organs. In the proximal portion of each, the individual segments are short and wider than long, and in the distal region they are narrow and longer than wide.
There are four pairs of biramous cephalic appendages, which differ only very slightly from the appendages of the thorax. All are of course excessively flattened, and they are here described as they appear.
The coxopodites, shown for the first time in Walcott's paper of 1918, are broad, longer than wide, and truncated on the inner ends, where they bear short, stout, unequal spines [22] similar to those along the anterior margin. The gnathobases are but slightly modified to serve as mouth parts, much less so than in Triarthrus, but the coxopodites of the cephalon are shorter and wider than those of the thorax.
At the distal end of the coxopodite arise the endopodite and exopodite. The endopodite consists of six segments, the distal ones, propodite and dactylopodite, more slender than the others, the last bearing three terminal spines. The first endopodite is shorter than the others and slightly more slender (pl. 16, fig. 1)[1] and the anterior appendages turn forward more or less parallel to the sides of the hypostoma (pl. 22). The basipodite, ischiopodite, meropodite, and carpopodite are, in their flattened condition, roughly rectangular, only a little longer than wide, taper gradually distally, each bears small spines on the outer rim, and some of the proximal ones usually have a row along the margin.
[1] Nota bene! All references in this section are to the plates of Doctor Walcott's paper in 1918.
The exopodites of the cephalon, as of the body of Neolenus, are very different from those of any other trilobite whose appendages were previously known. As shown in the photographs (pl. 20, fig. 2; pl. 22), each exopodite consists of a single long, broad, leaf-like blade, not with many segments as in Triarthrus, but consisting of a large basal and small terminal lobe. It bears on its outer margin numerous relatively short, slender, flat setæ. The long axes of the exopodites point forward, and the setæ are directed forward and outward. They stand more nearly at right angles to the shaft on the cephalic exopodites than on those of the thorax. This same type of broad-bladed exopodite is also found on the thorax and pygidium.
The number of functional gnathobases on the cephalon is unknown. That four endopodites were present on one side is shown pretty clearly by specimen 58591 (pl. 16, fig. 3) and while no more than two well preserved exopodites have been seen on a side, there probably were four. Specimen 65513 (pl. 16, fig. 1) shows gnathobases on the second and third appendages of that individual as preserved, but there is no positive evidence that these are really the second and third appendages, for they are obviously displaced. The hypostoma of Neolenus is narrow but long, several specimens showing that it extended back to the horizon of the outer ends of the last pair of glabellar furrows. It is not as wide as the axial lobe, so that, while gnathobases attached beneath the first pair of furrows would probably not reach back to the posterior end of the hypostoma, they might lie parallel to it and not extend beneath. It seems possible, then, that there were four pairs of endobases but that the second rather than the first pair served as mandibles, as seems to be the case in Ceraurus.
The thorax of Neolenus consists of seven segments, and the appendages are well shown (pl. 17, fig. 1; pl. 18, figs. 1, 2; pl. 20, fig. 1.), The endopodites of successive segments vary but little, all are slender but compact, and consist of a long coxopodite with six short, rather broad segments beyond it. In the figures, the endopodites extend some distance in a horizontal direction beyond the edges of the dorsal test, as many as four segments being in some cases visible, but measurements show that the appendages tended to fall outward on decay of the animal. The dactylopodites are provided with terminal spines as in Triarthrus. The coxopodites are long, straight, and slender. They are well shown on only one specimen (pl. 18), where they are seen to be as wide as the basipodite, and the endobases are set with spines on the posterior and inner margins. They are so long that those on opposite [23] sides must have almost met on the median line. The segments of the endopodites are mostly but little, if any, longer than broad, and at the distal end each shows two or more spines. The propodite and dactylopodite are notably more slender than the others. The exopodites of the thorax are broad and flat, and each shaft has two distinct parts with different kinds of setæ. The posterior edge of the proximal lobe is fringed with a slender, flat, overlapping hairs which are a little longer than the width of the lobe, and stand at an angle of about 60 degrees with the direction of the axis of the appendage. The outer lobe is at an angle with the main one, and has short, very fine setæ oh the margin. One or two specimens show some evidence of a joint between the inner and outer lobes, but in the great majority of cases they seem to be continuous; if originally in two segments, they have become firmly united. The exopodites of the thorax, like those of the cephalon, are directed diagonally forward and outward. (pl. 21, fig. 6; pl. 22.)
The pygidium of Neolenus serratus is large, and usually shows five rings on the axial lobe and four pairs of ribs on the sides. There are five pairs of biramous appendages belonging to this shield, and behind these a pair of jointed cerci. That the number of abdominal appendages should correspond to the number of divisions of the axial lobe rather than to the number of ribs on the pleural lobes is of interest, and in accord with other trilobites, as first shown by Beecher.
The endopodites of the pygidium have the same form as those of the thorax, are long, and very much less modified than those of any other trilobite whose appendages are known. On some specimens, they extend out far beyond the dorsal test, so that nearly all the segments are visible (pl. 17, fig. 3; pl. 18; pl. 19; pl. 20, fig. 1), but in these cases are probably displaced. The segments are short and wide, the whole endopodite tapering gradually outward. The dactylopodite bears terminal spines, and the individual segments also have outward-directed spines.
The cerci appear to have been long, slender, very spinose organs much like the antennules, but stiff rather than flexible. They are a little longer than the pygidium (pl. 17, figs. 1, 2), and seem to be attached to a plate on the under surface of the posterior end and in front of the very narrow doublure. The precise form of this attachment can not be determined from the published figures. They bear numerous fine spines (pl. 17, fig. 3).
Doctor Walcott has found on several specimens of Neolenus remains of organs which he interprets as epipodites and exites attached to the coxopodites. A study of the specimens has, however, convinced me that both the large and small epipodites are really exopodites, and that the exites are badly preserved and displaced coxopodites. Detailed explanation of this interpretation is given below in the description of the several specimens involved.
Description of Individual Specimens.
Doctor Walcott was kind enough to send me eight of the more important specimens of Neolenus figured by him, and since my interpretation of them does not agree in all respects with his, I have thought it fairer to the reader to present here rather full notes explaining the position I have taken. I understand that since I communicated my interpretation of the epipodites and exites to him, Doctor Walcott has submitted the specimens to [24] several palæontologists, who consider that epipodites are really present. Since I am not able to convince myself that their conclusion is based upon sound evidence, I give here my own interpretation. There is of course, no a priori reason why trilobites should not have had epipodites.
Specimen No. 58589.
Illustrated: Walcott, Smithson. Misc. Coll., vol. 57, 1912, pl. 45, fig. 2;—Zittel-Eastman Text-book of Paleontology, vol. 1, 1913, fig. 1377;—Smithson. Misc. Coll., vol. 67, 1918, pl. 18, fig. 1; pl. 20, fig. 1.
This is one of the most important of the specimens, as it shows the coxopodites of three thoracic limbs and the well preserved endopodites of six thoracic and five pairs of pygidial appendages.
The appendages are all shifted to the left till the articular socket of the coxopodite is. about 8 mm. outside of its proper position. The endopodites extend a corresponding amount beyond the edge of the dorsal test and are there so flattened that they are revealed as a mere impression. The coxopodites, which are beneath the test, seem to have been somewhat protected by it, and while hopelessly crushed, are not flattened, but rather conformed to the ridges and grooves of the thorax.
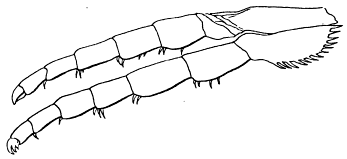
Fig. 2. Neolenus serratus (Rominger). A sketch of the coxopodites and endopodites of two thoracic segments. Note notch for the reception of the lower end of the appendifer. × 3.
The coxopodite of the appendage of the last thoracic segment is best preserved. It is rectangular, about one third as wide as long, with a slight notch in the posterior margin near the outer end. The inner end is obliquely truncated and shows about ten sharp spines which do not appear to be articulated to the segment, but rather to be direct outgrowths from it. There are similar spines along the posterior margin, but only two or three of what was probably once a continuous series are now preserved. On the opposite margin of the coxopodite from the slight depression mentioned above, there is a slight convexity in the outline, which is better shown and explained by the coxopodite just in front of this. That basal segment has the same form as the one just described, but as its posterior margin is for the greater part of its length pushed under the one behind it, the spines are not shown. On the posterior margin, two-thirds of the length from the proximal end, there is a shallow notch, and corresponding to it, a bulge on the anterior side. From analogy with Ceraurus and Calymene it becomes plain that the notch and bulge represent the position of the socket where the coxopodite articulated with the appendifer. Since these structures have not been shown in previous illustrations, a drawing giving my interpretation of them is here inserted [25] (fig. 2). It is evident from the position of the notch that the row of spines was on the dorsal (inner) side of the coxopodite and that the truncation was obliquely downward and outward.
The endopodite of the last thoracic appendage is well preserved and may be described as typical of such a leg in this part. The basipodite is as wide as the coxopodite, and it and the three succeeding segments, ischiopodite, meropodite, and carpopodite, are all parallel-sided, not expanded at the joints, and decrease regularly in width. The propodite and dactylopodite are also parallel-sided, but more slender than the inner segments, and on the end of the dactylopodite there are four little spines, three of them—one large and two small—articulated at the distal end, and the fourth projecting from the posterior outer angle. Each segment has one or more spines on the outer articular end, and the ischiopodite has several directed obliquely outward on the posterior margin. All of the four proximal segments show a low ridge parallel to and near the anterior margin, and several endopodites of the pygidium have a similar ridge and a row of spines along the posterior margin of some of the segments. These features indicate that the segments in question were not cylindrical in life, but compressed. From the almost universal location of the spines on the posterior side of the limbs as preserved, it seems probable that in the natural position the segments were held in a plane at a high angle with the horizontal, the ridge was dorsal and anterior and the row of spines ventral and posterior. Because the spines on the endobases are dorsal it does not follow that those on the endopodites were, for the position of the coxopodite in a crushed specimen does not indicate the position of the endopodite of even the same appendage.
The endopodites of the pygidium are similar to the one just described, except that some of them have spines on the posterior margin of the segments, and a few on the right side have extremely fine, faintly visible spines on the anterior side. The specimen shows fragments of a few exopodites, but nothing worth describing. In the middle of the right pleural lobe there is a small organ which Walcott has interpreted as a small epipodite. It is oval in form, broken at the end toward the axial lobe, and has exceedingly minute short setæ on the posterior margin. From analogy with other specimens, it appears to me to be the outer end of an exopodite.
Measurements: The entire specimen is about 64 mm. long and 52 mm. wide at the genal angles. The thorax is about 41 mm. wide (disregarding the spines) at the seventh segment, and the axial lobe about 13 mm. wide at the same horizon. The measurements of the individual segments of the seventh left thoracic limb are:
Coxopodite, 9 mm. long, 3 mm. wide, the middle of the notch 8 mm. from the inner end, measured along the bottom, and 6 mm. measured along the top. |
||||||
Basipodite, | 5 | mm. | long, | 3 | mm. | wide |
Ischiopodite, | 4 | " | " | 3 | " | " |
Meropodite, | 3.5 | " | " | 2.5 | " | " |
Carpopodite, | 3.5 | " | " | 2 | " | " |
Propodite, | 3 | " | " | 1.25 | " | " |
Dactylopodite, | 2 | " | " | 1.25 | " | " |
The five distal segments of the last pygidial endopodite are together 10.5 mm. long. The whole six segments of the endopodite of the third thoracic segments are together 21 mm. long. The distance from the appendifer of the third segment to the outer end of the spine is 17 mm. From the center of the notch in the coxopodite to the outer end is 1.5 mm., [26] which, added to the length of the endopodite, 21 mm., makes a distance of 22.5 mm. from the appendifer to the tip of the dactylopodite, showing that if projected straight outward, the endopodites of the thorax would project 5.5 mm. beyond the test, including spines.
The distance across the axial lobe from appendifer to appendifer on the seventh thoracic segment is 12.5 mm. Measured along the top of the coxopodite, it is 6 mm. from the middle of the notch to the inner end, and measured along the bottom it is 8 mm. From the truncated form of the ends it is evident that the coxopodites extended inward and downward from the appendifers, and with the dimensions given above, the inner toothed ends would practically meet on the median line.
Measurements on the appendages of the pygidia show that on this specimen they extend back about twice as far beyond the edge of the pygidium as they should, all being displaced.
Specimen No. 65514.
Illustrated: Walcott, Smithson. Misc. Coll., vol. 67, 1918, pl. 19, figs. 1-3.
This specimen is so twisted apart that it is not possible to determine to what segments the appendages belong, but it exhibits the best preserved exopodites I have seen. The best one is just in front of the pygidium on the matrix, and shows a form more easily seen than described (our fig. 3). There is a broad, flat, leaf-like shaft, the anterior side of which follows a smooth curve, while in the curve on the posterior side, which is convex backward, there is a re-entrant, setting off a small outer lobe whose length is about one third the length of the whole. This lobe seems to be a continuation of the shaft, and the test of the whole is wrinkled and evidently very thin. The main and distal lobes of the shaft both bear numerous delicate setæ, but those of the outer lobe are much shorter and finer than those on the main portion. The latter are flattened and blade-like.
![]() |
![]() |
Fig. 3. Exopodite of Neolenus serratus (Rominger), to show form of the lobes of the shaft, and the setæ. × 4. |
Fig. 4. Neolenus serratus (Rominger). One of the so-called epipodites of specimen 65515, showing that it has the same outline as an exopodite (compare figure 3) and fragments of setæ on the margin. × 3. |
The anterior edge of the shaft shows a narrow stiffening ridge and the setæ are but little longer than its greatest width. The second segment of the pygidium has another exopodite like this one, but shows faintly the line between the two lobes, as though there were two segments.
This specimen also shows some very well preserved endopodites, but they differ in no way from those described from specimen No. 58589. Walcott mentions two large epipodites projecting from beneath the exopodites. I judge that he has reference to the distal lobes of the exopodites, but as these are continuous with the main shaft, there can be no other interpretation of them than that which I have given above.
Measurements: The pygidium is 19 mm. long (without the spines) and about 34 mm. wide at the front. The exopodites show faintly beneath the pygidial shield, but their proximal ends are too indistinct to allow accurate measurement. Apparently they were just about long enough to reach to the margin of the shield. The best preserved one, that of the second segment in the pygidium, is about 11 mm. long, 2.5 mm. wide at the widest; the distal lobe is 2.5 mm. long, and the longest setæ of the main lobe 3.5 mm. long. The pleural lobe of the pygidium is just 11 mm. wide at this point.
The endopodites project from 8 to 12 mm. beyond the pygidium, showing about four segments.
The thoracic exopodite described above is 11 mm. long and 2.75 mm. wide at the widest part. The distal lobe is 3.5 mm. long and 2.25 mm. wide, and the longest setæ on the main lobe 3 mm. long.
Specimen No. 65519.
Illustrated: Walcott, Zittel-Eastman Text-book of Paleontology, vol. 1, 1913, fig. 1343;—Smithson. Misc. Coll., vol. 67, 1918, pl. 21, fig. 6.
This specimen is somewhat difficult to study but is very valuable as showing the natural position of the exopodites of the anterior part of the thorax. Walcott's figures are excellent and show the broad leaf-like shafts, the distal lobes with the re-entrant angles in the posterior margin, and the long fine setæ of the main lobes. None of the distal lobes retains its setæ. All extend back to the dorsal furrows, but the proximal ends are not actually shown.
The specimen is especially important because it shows the same distal lobes as specimen No. 65514, and demonstrates that they are a part of the exopodite and not of any other structure.
Measurements: The exopodite belonging to the fourth thoracic segment is 23 mm. long and 4 mm. wide at the widest part. The longest setæ are 7 mm. in length.
Specimen No. 65520.
Illustrated: Walcott, Smithson. Misc. Coll., vol. 67, 1918, pl. 20, fig. 2; pl. 22, fig. 1.
This is a practically entire specimen, on two blocks, one showing the interior of the shell, and the other the one figured by Walcott, a cast of the interior. The first shows the low rounded appendifers at the anterior angle of each axial tergite. They are almost entirely beneath the dorsal furrows and do not project so far into the axial lobe as those of Ceraurus and Calymene. In fact, only those at the anterior end of the thorax project inward at all. As expected, there are five pairs on the pygidium. The cephalon is unfortunately so exfoliated that the appendifers there are not preserved. The doublure of the pygidium is extremely narrow.
The cast of the interior shows, rather faintly, the exopodites of the right side of the thorax and of the left side of the cephalon, and, still more faintly, the caudal rami and a few pygidial endopodites. The exopodites on the right side are in what seems to be the customary position, directed obliquely forward and outward, and the tips of their distal lobes project slightly beyond the edge of the test. These lobes were interpreted by Walcott as epipodites, but after comparing them with the terminal lobes of the exopodites of specimens No. 65519 and 65514 I think there can be no doubt that they represent the same structure. The pleura of the individual thoracic segments on this side of the specimen have an unusual appearance, for they are bluntly rounded or obtusely pointed, instead of being spinose.
The interpretation of the appendages of the cephalon is somewhat difficult. At the left of the glabella there are two large exopodites, the anterior of which lies over and partially conceals the other. These show by their position that they belong to the fourth and fifth cephalic appendages. In front of these lie two appendages which may be either endopodites or exopodites, but which I am inclined to refer to the latter. Both are narrow and shaped like endopodites, but bear on their outer edges close-set fine setæ. They also show what might be considered as faint traces of segmentation. If the first of these ran under the end of the exopodite behind it, as shown in Walcott's figure (pl. 22), then it would be necessary to interpret it as an endopodite, but it really continues down between the exopodite and the glabella, and seems to be attached opposite the middle of the eye. The specimen does not indicate clearly whether this appendage is above or below the exopodite behind it, but one's impression is that it is above, in which case it also must be an exopodite. The appendage in front, being similar, is similarly interpreted. If this be correct, then the exopodites of the second and third cephalic appendages are much shorter and narrower than those of the fourth and fifth. All of these appendages are obviously out of position, for the cheek has been pushed forward away from the thorax, though still pivoting on its inner angle at the neck-ring, till the eye has been brought up to the dorsal furrow. In this way the anterior exopodites have been thrust under the glabella and all the appendages have been moved to the right of their original position. The anterior exopodite is very poorly shown, but seems to be articulated in front of the eye. The posterior exopodites are very similar to those on the thorax. The distal lobe is shown only by the second from the last. It has the same form as the distal lobes on the thoracic exopodites, and like them has much finer setæ than the main lobe, but it does not stand at so great an angle with the axis of the main lobe, nor yet is it so straight as shown in Walcott's figure.
Measurements: The specimen is about 72 mm. long and 54 mm. wide at the genal angles. The pygidium is 22 mm. long and 37 mm. wide. The doublure is 1.5 mm. wide. The exopodite of the third thoracic segment is 19.5 mm. long. The pleural lobe at this point is 13 mm. wide without the spines and 18.5 mm. wide with them. The third exopodite of the cephalon was apparently about 15 mm. long when complete.
Specimen No. 65515.
Illustrated: Walcott, Smithson. Misc. Coll., vol. 67, 1918, pl. 20, figs. 3, 4.
This is a small piece of the axial portion of a badly crushed Neolenus, showing appendages on the left side as viewed from above. On the posterior half there are three large appendages which have the exact form of the exopodites of other specimens. There is a broad, oval, proximal lobe and a distal one at an angle with it. The proximal part of the shaft has fine setæ or the bases of them, and the distal lobe faint traces of much finer ones. The form, and the setæ so far as they are preserved, are exactly like those of the exopodites on the specimens previously described. (See fig. 4, page 26.) Beneath them there are slender, poorly preserved endopodites.
In front of the exopodites and endopodites lie a series of structures which Walcott has called exites, but for which I can see another explanation. Walcott has shown them as four broad rounded lobes, but his figure must be looked upon as a drawing and not as a photograph, for it has been very much retouched.
For convenience of discussion, these lobes may be called Nos. 1, 2, 3, and 4, the last being the posterior one (fig. 5). This lobe is best shown on the matrix, where the anterior end is seen to be margined by stout spines, while the posterior end lies over the endopodite and under the exopodite behind it. No. 3 is sunk below the level of the others, and only a part of it has been uncovered. Its margin bears strong spines of different sizes. Its full shape can not be made out, but it has neither the shape nor the form of spines shown in figure 3, plate 20 (1918). Lobes 2 and 1 and another lobe in front of 1 seem to form a continuous series and to be part of a single appendage. They are all in one plane, arc so continuous that the joints between them can be made out with difficulty and if they do belong together, can easily be explained.
Before calling these structures new organs not previously seen on trilobites, it is of course necessary to inquire if they can be interpreted as representing any known structures. That they can not be exopodites is obvious, since they are bordered by short stout spines instead of setæ. The same stout spines that negate the above possible explanation at once suggest that they are coxopodites (compare fig 6). At first sight, the so-called exites seem too wide and too rounded to be so interpreted, but if reference be had to the specimens rather than the figures, it will be noted that the only well preserved structure (No. 2) is longer than wide, has spines only on one side and one end, and does not differ greatly from the coxopodite of specimen No. 58589 (pl. 18, 1918). If structures 2, 1, and the segment ahead of 1 are really parts of one appendage, it can only be an endopodite, of which No. 2 is the coxopodite, No. 1 the basipodite, and the next segment the ischiopodite. If one looks carefully, there are no traces of spines on either end of No. 1, but only on the margin. The extreme width of No. 2 is against this interpretation as a coxopodite (see, however, fig. 6), but it may be rolled out very flat, as this is an unusually [30] crushed specimen. No. 2 is 10 mm. long and 6 mm. wide at the widest point. No. 1 is 5 mm. long and 3.5 mm. wide.
The crucial point in this determination is whether 2 and 1 are parts of the same appendage. I believe they are, but others may differ.
Specimen No. 65513.
Illustrated: Walcott, Smithson. Misc. Coll., vol. 57, 1912, pl. 45, fig. 3;—Ibid., vol. 67, 1918, pl. 16, figs. 1, 2.
This is nearly all of the right half of an entire specimen, but the only appendages of any interest are those of the cephalon. Five endopodites emerge from beneath that shield, but as all are displaced it is not possible to say how many belong to the head. When held at the proper angle to the light, the second and third from the front show faintly the partial outlines of the coxopodites. The anterior side and end of the best preserved one shows irregular stout spines of unequal sizes, and the inner end is truncated obliquely (fig. 6). These coxopodites are like those on the thorax of specimen No. 58589, but shorter and wider. This of course suggests that the "exite" No. 2 of specimen No. 65515 may be a cephalic coxopodite. The endopodite of this appendage, like the others on this cephalon, is shorter and stouter than the thoracic or pygidial endopodites of the others described.
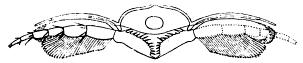
Fig. 7.—A restored section across the thorax of Neolenus serratus, showing the probable form of attachment of the appendages, their relation to the ventral membrane, and the jaw-like endobases of the coxopodites.
Measurements: The cephalon is 24 mm. long and about 60 mm. wide. The coxopodite of the third appendage is about 10 mm. long and 5.5 mm. wide at the widest point. The corresponding endopodite is 19 mm. long and projects 11 mm. beyond the margin, which is about 5 mm. further than it would project were the appendage restored to its proper position.
This restoration is based upon the information obtained from the studies which have been detailed in the preceding pages, and differs materially from that presented by Doctor Walcott. The appendages are not shown in their natural positions, but as if flattened nearly into a horizontal plane. The metastoma is added without any evidence for its former presence.
The striking features of the appendages are the broad unsegmented exopodites which point forward all along the body, and the strong endopodites, which show practically no regional modification. Although the exopodites have a form which is especially adapted for use in swimming, their position is such as to indicate that they were not so used. The stout endopodites, on the other hand, probably performed the double function of natatory and ambulatory legs.
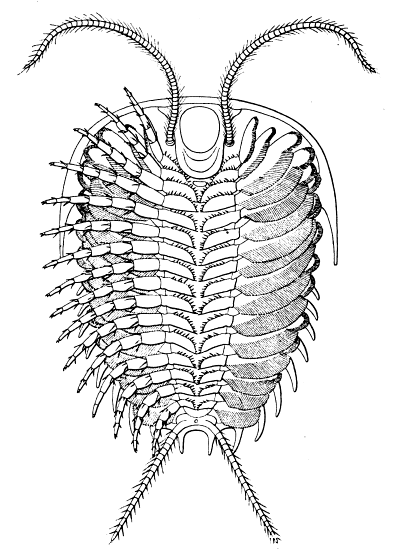
Fig. 8.—Neolenus serratus (Rominger). A restoration of the ventral surface, with the endopodites omitted from one side, to permit a better exposition of the exopodites. The position and number of the appendages about the mouth are in considerable doubt. Restored by Doctor Elvira Wood under the supervision of the writer. About one-half larger than the average specimen.
Nathorstia transitans Walcott.
Illustrated: Walcott, Smithson. Misc. Coll., vol. 57, 1912, pl. 28, fig. 2.
The badly preserved specimen on which this genus and species was based is undoubtedly a trilobite, but for some reason it does not find a place in Walcott's recent article on "Appendages" (1918). The preservation is different from that of the associated trilobites, being merely a shadowy impression, indicating a very soft test. The general outline [32] of the body, the position of the eye, and even a trace of spines about the pygidium (in the figure) are similar to those of Neolenus, and I would venture the suggestion that Nathorstia transitans is a recently moulted Neolenus serratus, still in the "soft-shelled" condition. Even if not a Neolenus, it is probable, from the state of preservation, that it is an animal which had recently cast its shell.
Walcott describes such fragments of appendages as remain, as follows:
Head. A portion of what may be an antenna projects from beneath the right anterior margin; from near the left posterolateral angle a large four-jointed appendage extends backward. I assume that this may be the outer portion of the large posterior appendage (maxilla) of the head.
Thorax. Traces of several slender-jointed thoracic legs project from beneath the anterior segments and back of these on the right side more or less of six legs have been pushed out from beneath the dorsal shield; these are composed of three or four long slender joints; fragments of the three proximal joints indicate that they are shorter and larger and that they have a fringe of fine setæ. Indications of a branchial lobe (gill) are seen in two specimens where the legs are not preserved. This is often the case both among the Merostomata (pl. 29, fig. 3, Molaria) and Trilobita (pl. 24, fig. 2, Ptychoparia).
Two caudal rami project a little distance beneath the posterior margin of the dorsal shield.
This latter feature of course suggests Neolenus. The other appendages are too poorly preserved to allow comparison without seeing the specimen.
The specific name was given "on account of its suggesting a transition between a Merostome-like form, such as Molaria spinifera, and the trilobites." In what respect it is transitional does not appear.
Formation and locality: Same as that of Neolenus serratus. One nearly complete specimen and a few fragments were found.
The Appendages of Isotelus.
The first specimen of Isotelus with appendages was described orally by Billings before the Natural History Society of Montreal in 1864, and in print six years later (1870, p. 479, pls. 31, 32). The specimen is described in detail on a later page. Billings recognized the remains of eight pairs of legs on the thorax, a pair for each segment, and he inferred from the fact that the appendages projected forward that they were ambulatory rather than natatory organs. He was unable to make out the exact number of the segments in the appendages, but thought each showed at least four or five.
Having examined the individual sent to London by Billings, Woodward (1870, p. 486, fig, 1) reviewed the collection from the American Trenton in the British Museum and found a specimen in the "Black Trenton limestone," from Ottawa, Ontario, in which, alongside the hypostoma, was a jointed appendage, which he described as the "jointed palpus of one of the maxillæ." This has always been considered an authentic "find," but I am informed by Doctor Bather that the specimen does not show any real appendage. For further discussion, see under Isotelus gigas.
In 1871, Billings' specimen was examined by Professors James D. Dana (1871, p. 320), A. E. Verrill, and Sydney I. Smith, who agreed that the structures identified by Billings as legs were merely semicalcified arches of the membrane of the ventral surface, which opinion seems to have been adopted by zoologists generally in spite of the fact that the most elementary consideration of the structure of the thorax of a trilobite should have shown its falsity. While the curvature of the thoracic segments was convex forward, that of the supposed ventral arches was convex backward, and the supposed arches extended [33] across so many segments as to have absolutely prevented any great amount of motion of the segments of the thorax on each other. Enrollment, a common occurrence in Isotelus, would have been absolutely impossible had any such calcified arches been present.
Walcott, in his study of trilobites in thin section (1881, pp. 192, 206, pl. 2, fig. 9), obtained eleven slices of Isotelus gigas which showed remains of appendages. He figured one of the sections, stating that it "shows the basal joint of a leg and another specimen not illustrated gives evidence that the legs extended out beneath the pygidium, as indicated by their basal joints."
The second important specimen of an Isotelus with appendages was found by Mr. James Pugh in strata of Richmond age 2 miles north of Oxford, Ohio, and is now in the U. S. National Museum. It was first described by Mickleborough (1883, p. 200, fig. 1-3). In two successive finds, a year apart, the specimen itself and its impression were recovered. Since I am redescribing the specimen in this memoir (see p. 35), it only remains to state here that Mickleborough interpreted the structures essentially correctly, though not using the same terminology as that at present adopted. His view that the anterior appendages were chelate can not, however, be supported, nor can his idea that the sole appendages of the pygidium were foliaceous branchial organs.
Walcott (1884, p. 279, fig. 1) studied the original specimens and presented a figure which is much more detailed and clear than those of Mickleborough. By further cleaning the specimen he made out altogether twenty-six pairs of appendages. He stated that one of these belonged to the cephalon, nine to the thorax,[1] and the remaining sixteen to the pygidium. He showed that the endopodites of the pygidium were of practically the same form as those on the thorax, and stated that the "leg beneath the thorax of the Ohio trilobite shows seven joints in two instances; the character of the terminal joint is unknown." His figure shows, and he mentions, markings which are interpreted as traces of the fringes of the exopodites.
[1] The posterior one of these he believed to have been crowded forward from beneath the pygidium.
In the same year Woodward (1884, p. 162, fig. 1-3) reproduced all of Mickleborough's figures, and suggested that the last seven pairs of appendages on the pygidium of Calymene and Isotelus were probably "lamelliform branchiferous appendages, as in Limulus and in living Isopoda."
Professor Beecher published, in 1902, an outline taken from Mickleborough's figure of this specimen, to call attention to certain discontinuous ridges along the axial cavity of the anterior part of the pygidium and posterior end of the thorax. These ridges are well shown in Mickleborough's figure, though not in that of Walcott, and their presence on the specimen was confirmed by a study by Schuchert, who contributed a diagrammatic cross-section to Beecher's paper (1902, p. 169, pl. 5, figs. 5, 6). Beecher summarized in a paragraph his interpretation of this specimen:
The club-shaped bodies lying within the axis are the gnathobases attached at the sides of the axis; the curved members extending outward from the gnathobases are the endopodites; the longitudinal ridges in the ventral membrane between the inner ends of the gnathobases are the buttresses and apodemes of the mesosternites; the slender oblique rod-like bodies shown in the right pleural region in Walcott's figure are portions of the fringes of the exopodites.
In 1910, Mr. W. C. King of Ottawa, Ontario, found at Britannia, a few miles west of Ottawa, the impression in sandstone of the under surface of a large specimen of Isotelus arenicola, described on a later page (p. 39).
Finally (1918, p. 133, pl. 24, figs. 3, 3a; pl. 25), Walcott has redescribed the specimen from Ohio, presenting a new and partially restored figure. He refers also to the specimen from Ottawa under the name Isotelus covingtonensis? Foerste (not Ulrich). He advances the view, which I am unable to share, that the cylindrical appearance of the segments of the appendages of Isotelus is due to post-mortem changes.
Illustrated: Asaphus platycephalus Billings, Quart. Jour. Geol. Soc., London, vol. 26, 1870, pl. 31, figs. 1-3; pl. 32, figs. 1, 2.—Woodward, Geol. Mag., vol. 8, 1871, pl. 8, figs. 1, 1a.—Gerstäcker, in Bronn's "Klassen u. Ordnungen d. Thier-Reichs," 1879, pl. 49, fig. 1.—von Koenen, N. Jahrb. f. Min., etc., vol. 1, 1880, pl. 8, fig. 8.—Milne-Edwards, Ann. Sci. Nat., Zoologie, ser. 6, vol. 12, 1881, pl. 12, fig. 45.
Isotelus latus Raymond, Bull. Victoria Mem. Mus., Geol. Survey Canada, No. 1, 1913, p. 45 (species named).
Isotelus covingtonensis? Walcott (not Foerste), Smithson. Misc. Coll., vol. 67, 1918, p. 134.
Knowledge of the appendages of this species is derived from the specimen which Billings described in 1870. It was found in the Trenton, probably the Middle Trenton, near Ottawa, Ontario, and is preserved in the Victoria Memorial Museum at Ottawa.
Viewed from the upper surface, it shows a large part of the test, but is broken along the sides, so that parts of the free cheeks, considerable of the pleural lobes of the thorax, and one side of the pygidium are missing. Viewed from the lower surface, the appendages are practically confined to the cephalon and thorax.
A short time before his death, Professor Beecher had this specimen and succeeded in cleaning away a part of the matrix so that the appendages show somewhat more clearly than in Billings' time, but they are not so well preserved as on the Mickleborough specimen, found in Ohio somewhat later.
The hypostoma is in place and well preserved; the posterior points are but 3 mm. in advance of the posterior margin of the cephalon. Behind the hypostoma there are only two pairs of cephalic appendages, the first of which is represented by the coxopodite and a trace of the endopodite. The outer end of the coxopodite is close to the outer margin of one of the prongs of the hypostoma and about 3 mm. in front of its posterior end. The gnathobase curves backward and inward, and appears to pass under the tip of the hypostoma. There were probably two appendages in front of this, whose gnathobases projected under the hypostoma, but the specimen shows nothing of them unless it be that one small fragment about 2 mm. back of the center is really a part of a gnathobase.
The specimen retains only the coxopodite and basipodite of the posterior cephalic appendage on the left side. The coxopodite is long and apparently cylindrical, the cross-section being of uniform diameter throughout the length. The inner portion is nearly straight, while the outer part is curved gently forward.
It is possible to make out remains of eight pairs of appendages on the thorax, some of them represented by coxopodites only, but most with more or less poorly preserved endopodites as well. No exopodites are visible. The coxopodites of the thorax seem to be of the same form as the last one on the cephalon, but slightly less curved. All are long and heavy, and there seems to be no decrease in size toward the pygidium. The endopodites are very imperfectly shown. They seem to be longer than those of Isotelus maximus, and the segments, while of less diameter than the coxopodites, do not show so great a contrast to [35] them as do those of that species. The direction of the endopodites is diagonally forward, and the outer portions do not appear to be curved backward as in Isotelus maximus. It would appear also that the endopodites were nearly or quite long enough to reach the outer margin of the dorsal test. On no endopodite can more than three segments be definitely distinguished, but the longest ones are the most obscurely segmented.
No appendages are preserved on the pygidium, but at one side of the median groove there are two projections which may be processes to which the appendages were attached.
Measurements: Total length of specimen, 109 mm. Probable length when complete, 116 mm. Length of cephalon, 40 mm.; width at genal angles, restored, about 62 mm. (Billings' restoration). Width of doublure of front of cephalon on median line, 17 mm.; length of hypostoma, 20 mm. Length of coxopodite of last appendage on left side of cephalon, 10.5 mm.; length of basipodite of the same appendage, 5 mm. Diameter of coxopodite, 2 mm.; diameter of basipodite, 1.5 mm. Length of coxopodite on left side of the second segment of the thorax, 11 mm.; diameter, about 2.5 mm. Length of basipodite of the same, 5 mm.; diameter, about 1.5 mm. Length of ischiopodite, 3.5 mm.; diameter, about 1.5 mm. Length of meropodite, 2.5 mm. (this may be less than the total length as the segment is not completely exposed.) Distance between proximal ends of gnathobases of the fifth thoracic segment, about 7 mm. Distance between outer ends of the coxopodites of the first thoracic segment (estimated from measurements on the left side), 27 mm Distance apart of the dorsal furrows at the first thoracic segment, 27 mm. Length of the longest exopodite which can be traced, about 20 mm.
Illustrated: Mickleborough, Jour. Cincinnati Soc. Nat. Hist., vol. 6, 1883, p. 200, figs. 1-3 (endopodites and coxopodites). Walcott, Science, vol. 3, 1884, p. 279, fig. 1 (endopodites, coxopodites, and traces of exopodites). Woodward, Geol. Mag., dec. 3, vol. 1, 1884, p. 162, figs. 1-3 (copies of Mickleborough's figures). Bernard, The Apodidæ, 1892, text fig. 49. Beecher, Amer. Jour. Sci., vol. 13, 1902, p. 169, pl. 5. figs. 5, 6 (outline from one of Mickleborough's figures and an original figure). Walcott, Smithson. Misc. Coll., vol. 67, 1918, p. 133, pl. 24, figs. 3, 3a; pl. 25, fig. 1.
This specimen, which conies from the Richmond strata 2 miles north of Oxford, Ohio, is the best preserved of the specimens of Isotelus with appendages which has so far been found. The individual consists of two parts, the actual specimen, and the impression of the ventral side.
To describe it I am using very skillfully made plaster reproductions of both parts, presented to the Museum of Comparative Zoology by Doctor Charles D. Walcott, and presumably made after he cleaned the specimen as described in Science (1884). I have also an enlarged photograph (pl. 10, fig. 2) which seems to have been made after some later period of cleaning, probably by Professor Beecher, and I have examined the original specimens in Washington.
Viewed from the dorsal side, it is seen that the individual is very imperfect, the greater part of the cephalon being removed by a diagonal break which cuts off the anterior third of the left eye and extends to the front of the second thoracic segment on the right side. The ends of the pleura of both sides of the thorax are broken away, as are also the greater parts of the pleural lobes and the posterior end of the pygidium. On the ventral side, merely the posterior tips of the hypostoma remain, but the distal ends of the appendages were so far within the outer margin that the appendagiferous area is quite fully retained.
The most conspicuous feature of this specimen is the presence of nine pairs of large coxopodites behind the hypostoma, and of the remains of ten pairs of endopodites, making in all ten pairs of appendages which are easily seen. The apportionment of these segments to cephalon, thorax, and pygidium is not agreed upon by the people who have examined the specimens, but if one remembers that it is the outer and not the inner end of the coxopodite which articulates with the appendifer, it at once becomes evident that the first two pairs of appendages on the specimen are the last two pairs belonging to the cephalon, and that the next eight pairs are those of the thorax.
The impressions of fourteen pairs of coxopodites are readily counted on the pygidium, and as Doctor Walcott noted sixteen pairs on the actual specimens, his number was probably correct.
Projecting the line of the back of the cephalon through from the dorsal side, it is found that the posterior tips of the hypostoma are 7 mm. in front of the posterior margin of the cephalon, and that the points of attachment of the posterior pair of cephalic appendages (the second pair shown on the specimen) are just within the posterior margin. The gnathobases of this pair of appendages extend back some distance beneath the thorax, and so give the impression that they belong to that part of the body. So far as can be determined, the cephalic appendages do not differ in any way from those of the thorax. On the mould of the ventral surface, just outside of the lateral edge of the right lobe of the hypostoma, is the somewhat imperfectly shown impression of the endopodite of the third cephalic appendage. The point of junction of the endopodite and coxopodite is about 2 mm. in front of the tip of the adjacent branch of the hypostoma, and the gnathobase is curved around just behind it. This accounts for three of the pairs of cephalic appendages. The second cephalic appendages must have thrust their gnathobases under the prongs of the hypostoma, and the endopodites were probably close to its edge. No trace of this pair appears on the specimen.
The thoracic appendages are the best preserved of any, and show the large coxopodites and the more slender endopodites which do not extend to the outer margin of the test. The latter extend forward and outward for about one half their length, then turn backward in a graceful curve.
Walcott's figure in Science shows hair-like markings on the under side of the right half of the thorax. These were interpreted by both Walcott and Beecher as fringes of the exopodites, but since the setæ of those organs on all other trilobites are always above the endopodites, while these are represented as below them, it would seem doubtful if this interpretation can be sustained. Furthermore, I find no trace of them on either cast or mould, and the actual specimen does not now show them.
The coxopodites and endopodites of the pygidium seem to be similar to those on the thorax, but both are shorter and more slender, and the former decrease in length rapidly toward the posterior end. As mentioned above, it is not perfectly plain how many appendages are present, but I have accepted Doctor Walcott's count of sixteen pairs. Of the endopodites only the barest traces are seen, and of exopodites nothing.
One point of considerable interest in this specimen is the thickness, as it probably gives some measure of the space occupied by the animal. In Triarthrus and other trilobites from Rome, New York, the appendages are pressed directly against the dorsal test, but in this specimen a considerable space intervenes between the plane of the appendages and the shell. Between the central furrow and the inner surface of the dorsal test at the anterior end of the thorax is a distance of 13 mm. and under the dorsal furrows the thickness is about 7 or 8 mm., no accurate measurement being possible in the present state of the specimen.
Measurements: Length of specimen on median line, 121 mm.; probable original length, about 195 mm. (Walcott's restoration). Length of thorax, 58 mm.[1] Width of axial lobe at the first thoracic segment, 45 mm.; total width as preserved, 92 mm.; width as estimated from the mould of the ventral surface, no mm.; Walcott's restoration, 105 mm.
[1] If this specimen had the same proportions as specimens of Isotelus maximus from Toronto, the total length would be only 174 mm. The cephalon would be about 52 mm. long, the thorax 58 mm., and the pygidium about 64 mm. long.
Length of coxopodite of fourth left cephalic appendage, about 18 mm.; diameter, about 2.5 mm. Length of coxopodite of last left cephalic appendage, about 18.5 mm. Distance apart of inner ends of gnathobases of fourth cephalic appendages, about 4 mm. Distance apart of inner ends of endobases of first thoracic segment, about 6 mm. Distance apart of outer ends of coxopodites of first thoracic segment, about 43 mm.
Length of coxopodite of seventh left thoracic appendage 16 mm., diameter about 3.5 mm.; length of basipodite of the endopodite of the same appendage 6 mm.; diameter about 2 mm.; length of ischiopodite 5 mm.; length of meropodite 4.5 mm.; length of carpopodite 4.5 mm.; length of propodite 3 mm.; length of dactylopodite 2.75 mm.; total length of endopodite 25.75 mm.
Length of coxopodite of fourth left thoracic appendage 20 mm., diameter 4 mm.; length of five proximal joints of the endopodite 25 mm.; diameter of basipodite about 2 mm.
(Text fig. 9.)
The exopodites have been omitted from this restoration since nothing is known of their actual form. The chief reason for the figure is to contrast the greatly developed coxopodites of the posterior part of the cephalon and thorax with those of other trilobites. The antennules and first two pairs of biramous appendages of the cephalon are more or less hypothetical, and less is known of the appendages of the pygidium than is shown here. The restoration is based somewhat upon Walcott's figure in Science. The outline is that of a specimen of Isotelus maximus from Toronto, Ontario.
Illustrated: Woodward, Quart. Jour. Geol. Soc., London, vol. 26, 1870, text fig. 1; Geol. Mag., dec. 3, vol. 1. 1884, p. 78, text fig. Milne-Edwards, Ann. Sci. Nat, Zoologie, ser. 6, vol. 12, 1881, pl. 12, fig. 46. Walcott, Bull. Mus. Comp. Zool., Harvard Coll., vol. 8, 1881, pl. 2, fig. 9; Geol. Mag., dec. 4, vol. 1, 1894, pl. 8, fig. 9; Proc. Biol. Soc. Washington, vol. 9, 1894, pl. 1, fig. 9.
The specimen in the British Museum which Woodward called Asaphus platycephalus, is, in all probability, an Isotelus gigas. Woodward says of it:
I was at once attracted by a specimen of Asaphus, from the Black Trenton Limestone (Lower Silurian), which has been much eroded on its upper surface, leaving the hypostoma and what appear to be the appendages belonging to the first, second, and third somites, exposed to view, united along the median line by a longitudinal ridge. The pseudo-appendages, however, have no evidence of any articulations. But what appears to me to be of the highest importance, as a piece of additional information afforded by the Museum specimen, is the discovery of what I believe to be the jointed palpus of one of the maxillæ, which has left its impression upon the side of the hypostoma—just, in fact, in that position which it must have occupied in life, judging by other Crustaceans which are furnished with an hypostoma, as Apus, Serolis, etc.
The palpus is 9 lines in length, the basal joint measures 3 lines, and is 2 lines broad, and somewhat triangular in form.
There appear to be about 7 articulations in the palpus itself, above the basal joint, marked by swellings upon its tubular stem, which is 1 line in diameter.
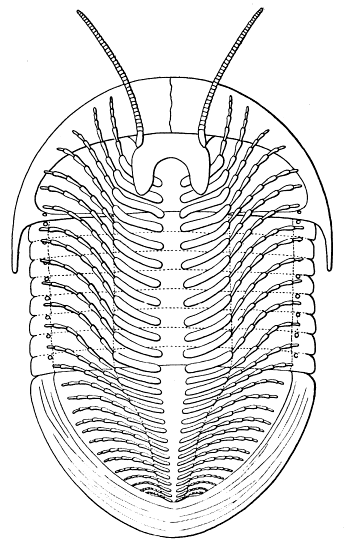
Fig. 9.—A restored composite of Isotelus maximus and I. latus. The exopodites are left out because entirely unknown. Drawn by Doctor Elvira Wood. Natural size.
Desiring to know more of this individual, I wrote to Doctor Bather and was surprised to learn that the specimen which was the basis of Woodward's observations is so badly preserved as to be of no real value. With his permission, I append a note made by Doctor Bather some years ago when selecting fossils to be placed on exhibition:
Asaphus gigas Dekay. Ordovician, Trenton Limestone. N. America, Canada. Descr. H. Woodward, 1870, Q. J. G. S., XXVI, pp. 486-488, text fig. 1, as Asaphus platycephalus. Coll. and presd. J. J. Bigsby, 1851. Regd. I 14431.
This specimen is in the Brit. Mus. Geol. Dept. I 14431. The supposed hypostome is exceedingly doubtful; it lies dorsad of the crushed glabellar skeleton. The "appendage" is merely the edge of a part in the head-shield; the maxilla is some calcite filling, between two such laminæ.
Walcott figured a slice of Isotelus gigas from Trenton Falls, New York, which shows a few fragments of appendages, but is of particular importance because it shows the presence of well developed appendifers beneath the axial lobe.
Illustrated: Ottawa Nat, vol. 24, 1910, p. 129, pl. 2, fig. 5.
The following quotations from my paper are inserted here to complete the record of appendage-bearing specimens:
A rather remarkable specimen of this species was found by W. C. King, Esq., on the shore of Lake Deschenes at Britannia [near Ottawa, Ontario]. This specimen is an impression of the lower surface of the trilobite, and shows a longitudinal ridge corresponding to the central furrow along the axis of the ventral side of the animal, ten pairs of transverse furrows, and the impression of the hypostoma. The doublure of the pygidium has also left a wide smooth impression, but in the cephalic region the hypostoma is the only portion of which there are any traces remaining. The specimen was found on a waterworn surface of the beach, partially covered by shingle….
The transverse furrows are the impressions left by the gnathobases of the basal joints of the legs. They were evidently long and very heavy, but the specimen has been so abraded that all details are obscured. The first six pairs of impressions are longer and deeper than the four behind. The first eight pairs seem to pertain to the thoracic appendages, while the last two belong to the pygidium. From the posterior tips of the hypostoma to the first gnathobases of which traces are present there is a distance of about 22 mm. without impressions. In Isotelus gigas the hypostoma normally extends back to the posterior margin of the cephalon, so that it seems that in this specimen the impressions of the first two pairs of gnathobases under the thorax may not have been preserved. In that case, the six pairs of strong impressions may represent the last six pairs of thoracic segments, and the pygidium might begin with the first of the fainter ones.
Horizon and locality: From the sandstone near the base of the Aylmer (Upper Chazy) formation at Britannia, west of Ottawa, Ontario. Specimen in the Victoria Memorial Museum, Geological Survey of Canada, Ottawa.
The Appendages of Triarthrus.
(Pls. 1-5; pl. 6, figs. 1-3; text figs. 1, 10, 11, 33, 42.)
(Also see Part IV.)
Illustrated: Matthew, Amer. Jour. Sci., vol. 46, 1893, pl. 1, figs. 1-7;—Trans. N. Y. Acad. Sci., vol. 12, pl. 8, figs. 1-7.—Beecher, Amer. Jour. Sci., vol. 46, 1893, text figs. 1-3;—Amer. Geol., vol. 13, 1894, pl. 3;—Amer. Jour. Sci., vol. 47, pl. 7, text fig. 1;—Amer. Geol., vol. 15, 1895, pls. 4, 5;—Ibid., vol. 16, 1895, pl. 8, [40] figs. 12-14; pl. 10. fig. 1;—Amer. Jour. Sci., vol. 1, 1896, pl. 8; Geol. Mag., dec. 4, vol. 3, 1896, pl. 9;—Eastman-Zittel Text-book of Paleontology, vol. 1, 1900, text figs. 1267-1269;—2d ed., 1913, fig. 1375; Studies in Evolution, 1901, reprint of all previous figs.;—Amer. Jour. Sci., vol. 13, 1902, pl. 2, figs. 1-5; pl. 3, fig. 1; pl. 4, fig. 1; pl. 5, figs. 2-4;—Geol. Mag., dec. 10, vol. 9, 1902, pls. 9-11, text figs. 1-3.—Walcott, Proc. Biol. Soc. Washington, vol. 9, 1894, pl. 1 figs. 1-6;—Geol. Mag., dec. 4, vol. 1, 1894, pl. 8;—Smithson. Misc. Coll., vol. 67, 1918, pl. 29, figs. 1-11; pl. 30, figs. 17-20; pl. 32; pl. 34, figs. 4-7; pl. 35, fig. 5.—Bernard, Quart. Jour. Geol. Soc., London, vol. 50, 1894, text figs. 11, 12.—Œhlert, Bull. Soc. Géol. France, ser. 3, vol. 24, 1896, text figs. 1-17, 34.—Jaekel, Zeits. d. d. geol. Gesell., vol. 53, 1901, text fig. 24. Moberg, Geol. Fören. Förhandl., vol. 29, pl. 5, 1907, pl. 4, fig. 2; pl. 5, fig. 1.—Handlirsch, Foss. Insekten, 1908, text fig. 6.—Tothill, Amer. Jour. Sci., vol. 42, 1916, p. 380, text fig. 5.—Crampton, Jour. N. Y. Entomol. Soc., vol. 24, 1917, pl. 2, fig. 20.
Historical.
Specimens of Triarthrus retaining appendages were first obtained by Mr. W. S. Valiant from the dark carbonaceous Utica shale near Rome, New York, in 1884, but no considerable amount of material was found until 1892. The first specimens were sent to Columbia University, and were described by Doctor W. D. Matthew (1893). This article was accompanied by a plate of sketches, showing for the first time the presence of antennules in trilobites and indicating something of the endopodites and exopodites of the appendages of the cephalon, thorax, and pygidium. Specimens had not yet been cleaned from the lower side, so that no great amount could then be learned of the detailed structure. Matthew concluded that "The homology with Limulus seems not to be as close in Triarthrus as in the forms studied by Mr. Walcott; but the characters seem to be of a more comprehensive type, approaching the general structure of the other Crustacea rather than any special form."
Professor Beecher's first paper, dated October 9, 1893, merely mentioned the fact that the Yale University Museum had obtained material from Valiant's locality, but was quickly followed by a paper read before the National Academy of Sciences on November 8, and published in December, 1893. This paper described particularly the thoracic appendages.
This was followed in January (1894 A) by an article in which some information about the mode of occurrence of the specimens was added, and in April (1894 B), the limbs of the pygidium were described and figured. The determination of the structure of the appendages of the head evidently presented some difficulty, for the article describing this portion of the animal did not appear until the next February (1895 A). This cleared up the ventral anatomy of Triarthrus, and was followed by a short article (1896 A) accompanied by a restoration of the trilobite showing all the appendages.
This ended Professor Beecher's publications on Triarthrus until his final paper in 1902, although he contributed some of his results and figures to his chapter on the trilobites in the Eastman-Zittel Text-book of Paleontology in 1900.
The discovery of these excellent specimens had of course excited very great interest. Doctor Walcott also studied a number of specimens from Valiant's locality, and published in 1894, with some original figures, the results of his comparison of the appendages of Triarthrus with those of Calymene and Ceraurus.
In his article on the "Systematic Position of the Trilobites," Bernard (1894) used the results of Professor Beecher's studies of 1893, and also quoted the papers by Matthew (1893) and Walcott (1894), though the article by the latter appeared too late to be used except for a note added while Bernard's paper was in press. A final footnote quoted from Professor Beecher's paper of April, 1894 (1894 B).
Œhlert (1896) gave an excellent summary in French of the work of Beecher and Walcott on Triarthrus, with reproductions of many of their figures.
Valiant (1901) in a non-technical article described his long search for trilobites with antennas. The discovery of the wonderful pyritized trilobites at Cleveland's Glen near Rome was not the result of a lucky accident, but the culmination of eight years of labor in a locality especially selected on account of the fineness of grain of the shale.
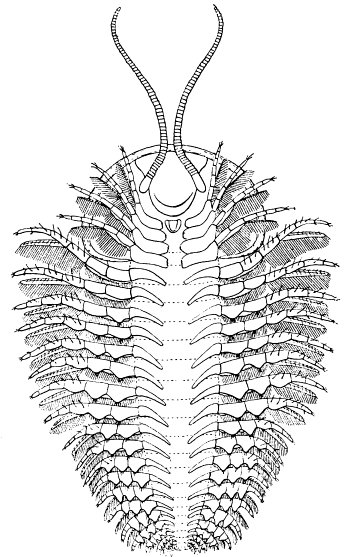
Fig. 10.—Triarthrus becki Green. A new restoration, modified from Professor Beecher's, to incorporate the results of his later work. The inner ends of the endobases are probably too far apart, as it was not discovered until after the drawing had been made that the appendifers projected within the dorsal furrows. Drawn by Doctor Elvira Wood. × about 3.8.
After 1896, Professor Beecher turned his attention largely to the problem of the classification of trilobites, and while he continued the arduous task of cleaning the matrix from [42] specimens of Triarthrus and Cryptolithus he did not again publish upon the subject of appendages until forced to do so by the doubts cast by Jaekel (1901) upon the validity of his earlier conclusions. Because of certain structures which he thought he had interpreted correctly from a poorly preserved specimen of Ptychoparia, Jaekel came to the conclusion that Beecher's material was not well preserved. Professor Beecher would have taken much more kindly to aspersions upon his opinions than to any slight upon his beloved trilobites, and his article on the "Ventral Integument of Trilobites" of 1902 was designed not only as an answer to Jaekel, but also to show by means of photographs the unusually perfect state of preservation of the specimens of Triarthrus. This article, like so many describing the appendages of trilobites, beginning with Matthew's, was published in two places (Beecher 1902).
Most of Beecher's papers, except the last one, were reprinted in the volume entitled "Studies in Evolution," published by Charles Scribner's Sons at the time of the Yale Bicentennial in 1901. The part pertaining particularly to Triarthrus is on pages 197 to 219.
Moberg (1907), in connection with a specimen of Eurycare angustatum which he thought preserved some appendages, described and illustrated some of the appendages of Triarthrus.
The most recent discussion of Triarthrus, with some new figures, is by Walcott (1918, p. 135, pls. 29, 30). He gives a summary of Beecher's work with numerous quotations. The principal original contribution is a discussion of the form and shape of the appendages before they were flattened out in the shale. He found also what he thought might possibly be the remains of epipodites on three specimens, one of which he illustrated with a photograph. I have seen nothing which could be interpreted as such an organ in the many specimens I have studied.
A point in which Walcott differs from Beecher in the interpretation of specimens is in regard to the development of the endopodites of small pygidia. Beecher (1894 B, pl. 7, fig. 3) illustrated a series of endopodites which he likened to the endites of a thoracic limb of Apus. Doctor Walcott finds that specimens in the United States National Museum show slender endopodites all the way to the back of the pygidium, and thinks that Beecher mistook a mass of terminal segments of exopodites for a series of endopodites. On careful examination, however, the specimen shows, as Beecher indicated, a series of endopodites in undisturbed condition (No. 222, our pl. 4, fig. 5).
One of the more important points noted in the later studies of Triarthrus is that the gnathites of the cephalic appendages are much less like the endobases under the thorax than Beecher earlier thought, and showed in his restored figures and in his model. The four gnathites of each side are curved, flattened, not club-shaped, and so wide and so close together that they overlap one another. The metastoma is somewhat larger and more nearly circular than Beecher's earlier preparations led him to suppose.
The restoration here presented is modified only slightly from the one designed by Professor Beecher, and the modifications are taken principally from figures published by him. The gnathites are drawn in form more like that shown by the specimens and his figures in the American Geologist (1895 A), and the metastoma is taken from one of the specimens. On the thorax the chief modification is in the addition of a considerable number of spines to the endopodites. In spite of the trivial character of most of these changes, they emphasize [43] one of the important characteristics of Triarthrus the regional differentiation of the appendages.
It should be pointed out that although Triarthrus is usually considered to be a very primitive trilobite, its appendages are more specialized than those of any of the others known. This is shown in their great length, the double curvature of the antennules, the differentiation of four pairs of endobases on the cephalon as gnathites, and the flattening of the segments of the posterior endopodites. These departures from the uniformity existing among the appendages of the other genera lead one to question whether the genus is really so primitive as has been supposed.
Relation of the Cephalic Appendages to the Markings on the Dorsal Surface of the Glabella.
Triarthrus becki is usually represented as having four pairs of glabellar furrows, but the two pairs at the front are exceedingly faint and the first of them is hardly ever visible, though that it does exist is proved by a number of authentic specimens. The neck furrow is narrow and sharply impressed, continuing across the glabella with a slightly backward curvature. In front of it are two pairs of linear, deeply impressed furrows which in their inward and backward sweep are bowed slightly forward, the ends of the corresponding furrows on opposite sides nearly meeting along the crest of the glabella. In front of these, near the median line, is a pair of slight indentations, having the appearance and position of the inner ends of a pair of furrows similar to those situated just behind them.
In front of and just outside this pair are the exceedingly faint impressions of the anterior pair of furrows, these, as said above, being but seldom seen. They are short, slightly indented linear furrows which have their axes perpendicular to the axis of the cephalon, and do not connect with each other or with the dorsal furrows. The latter are narrow, sharply impressed, and merge into a circumglabellar furrow at the front. In front of the circumglabellar furrow is a very narrow rounded ridge, but the anterior end of the glabella is very close to the margin of the cephalon.
Specimen No. 214, which was cleaned from the dorsal side, shows the posterior tip of the hypostoma, apparently in its natural position, 3.5 mm. back from the anterior margin. The entire length of the cephalon is 6 mm., so that the hypostoma reaches back slightly over one half the length (0.583). The greater part of it has been cleaned off, and one sees the proximal portions of the antennules, which are apparently attached just at the sides of the hypostoma, 2.5 mm. apart and 2.25 mm. back from the anterior edge of the cephalon. This position is distinctly within the outline of the glabella and corresponds approximately to the location of the second pair of glabellar furrows. Specimens 214, 215, 216, 217, and 219 all seem to show the same location for the bases of the antennules. Specimen 220 is the one in which the basal shafts are best preserved and the points of attachment seem to be further apart in it than in any of the others. This specimen is 38 mm. long, and the bases of the antennules are 5.5 mm. apart and 4 mm. behind the anterior margin. As the specimen is cleaned from the ventral side, the dorsal furrows do not show distinctly, but another specimen of about the same size (No. 228, 38.5 mm. long) has the dorsal furrows 8 mm. apart 4 mm. back of the anterior margin.
On the same slab with specimens 209 and 210 there is an individual which, although retaining the test, has had the proximal ends of the antennules so pressed against it that the course of the one on the left side is readily visible. It originates in a small oval mound [44] whose posterior margin impinges upon the third glabellar furrow near the middle of its course, and just outside the outer end of the second glabellar furrow. The cephalon of this specimen is 5 mm. long, and the point of origin of the left antennule is 2.75 mm. in front of the posterior margin and 0.75 mm. from the dorsal furrow.
It is therefore evident that the antennules in this species are not attached beneath the dorsal furrows, but within them and opposite the second pair of glabellar furrows.
All cephalic appendages behind the antennules are attached somewhat within the dorsal furrows, the first pair as far forward as the antennules and the last pair apparently under the anterior edge of the neck ring. They do not appear to correspond in position to the posterior glabellar furrows and neck ring, being more crowded. The last pair is attached to appendifers beneath the nuchal segment, and the first pair beneath the third glabellar furrows. There are no depressions on the dorsal surface corresponding to the points of attachment of the mandibles.
Professor Beecher, during his first studies of Triarthrus, found no appendages pertaining to the anal segment, but later evidently came upon a spinose anal plate which he caused to be figured. The specimen (No. 201) on which this appendage is preserved is cleaned from the dorsal side, and the anal plate is a small, bilaterally symmetrical, nearly semicircular structure margined with small spines. Specimen 202 also shows the same plate (pl. 5, fig. 6), but it is imperfectly preserved. It has a large perforation in the anterior half. Both of these specimens are in the Yale University Museum.
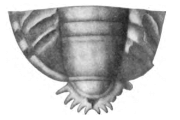
Fig. 11.—Triarthrus becki Green. Anal plate of specimen 65525 in the U. S. National Museum. Drawn by Doctor Wood. × 20.
The anal plate is especially well shown by specimen 65525 in the United States National Museum (fig. 11). This specimen is from Rome, New York, and two photographs of it have been published by Walcott (1918, pl. 29, fig. 6; pl. 30, fig. 19). It is developed from the dorsal side, and the anal plate is displaced, so that it projects behind the end of the pygidium. It is semicircular in shape, with a hemispheric mound at the middle of the anterior half. Two furrows starting from the anterior edge on either side of the mound border its sides, and, uniting back of it, continue as an axial furrow to the posterior margin. The mound is perforated for the opening of the posterior end of the alimentary canal. The lateral borders of the plate bear five pairs of short, symmetrically placed spines. The plate is 1 mm. wide and 0.5 mm. long, and the entire trilobite is 11.5 mm. long.
The Appendages of Ptychoparia.
Ptychoparia striata (Emmrich).
Illustrated: Jaekel, Zeits. d. d. geol. Gesell., 1901, vol. 53, part 1, pls. 4, 5.
Jaekel has described a specimen of this species obtained from the Middle Cambrian near Tejrovic, Bohemia, which on development showed beneath the test of the axial lobe, certain structures which he believed represented the casts of proximal segments of appendages. On the basis of this specimen he produced a new restoration of the ventral surface of the trilobite, in which he showed three short wide segments in the place occupied by the coxopodite of an appendage of Triarthrus. He also made the mouth parts considerably different from those of the latter genus. Beecher (1902) showed that the structures which Jaekel took for segments of appendages were really the fillings between stiffening plates of chitin on the ventral membrane, and demonstrated the fact that similar structures existed in Triarthrus. It cannot be said, therefore, that any appendages are really known in Ptychoparia striata, but some knowledge of the internal anatomy of the species is supplied by the specimen.
Ptychoparia cordilleræ (Rominger).
Illustrated: Walcott, Smithson. Misc. Coll., vol. 57, 1912, p. 192, pl. 24, fig. 2;—Ibid., vol. 67, 1918, pl. 21, figs. 3-5 (corrected figure).
Walcott has figured a single individual of this species showing appendages, the accompanying description being as follows (1918, p. 144):
Ventral appendages. Only one specimen has been found showing the thoracic limbs. This indicates very clearly the general character of the exopodite and that it is situated above the endopodite, although there are only imperfect traces of the latter….
The exopodites are unlike those of any trilobite now known. They are long, rather broad lobes extending from the line of the union of the mesosternites and the pleurosternites. At the proximal end they appear to be as wide as the axial lobe of each segment, and to increase in width and slightly overlap each other nearly out to the distal extremity…. They are finely crenulated along both the anterior and dorsal margins, which indicates the presence of fine setæ.
The specimen is quite imperfectly preserved, but seems to indicate that the exopodite of Ptychoparia had a long, rather narrow unsegmented shaft.
Measurements (from Walcott's figure): The specimen is a small one, about 9.5 mm. long, an individual exopodite is about 2 mm. long and the shaft 0.33 mm. wide.
Horizon and locality: Middle Cambrian, Burgess shale, between Mount Field and Wapta Peak, above Field, British Columbia.
Illustrated: Walcott, Smithson. Misc. Coll., vol. 67, 1918, p. 145, pl. 21, figs. 1, 2.
Walcott figured one individual of this species showing long slender antennules projecting in front of the cephalon. It is of especial interest because one of the antennules shows almost exactly the same sigmoid curvature which is so characteristic of the related Triarthrus. The individual segments are not visible.
Measurements: The specimen is 23 mm. long and the direct distance from the front of the head to the anterior end of the more perfect antennule is 9.5 mm. Measured along the curvature, the same antennule is about 11 mm. long.
Horizon and locality: Same as the preceding.
The Appendages of Kootenia.
Illustrated: Walcott, Smithson. Misc. Coll., vol. 67, 1918, pl. 14, figs. 2, 3.
One specimen figured by Doctor Walcott shows the distal ends of some of the exopodites and endopodites of the right side. He compares the exopodites with those of Neolenus, stating that the shaft consists of two segments, the proximal section being long and flat, fringed with long setæ, while the distal segment has short fine setæ. The endopodite best shown is very slender, and the segments are of uniform width and only slightly longer than wide.
Measurements (from Walcott's figures): Length of specimen, about 41 mm. Length of five distal segments of an endopodite, 7.5 mm. Since the pleural lobe is only 7 mm. wide, the endopodites, and probably the exopodites also, must have projected a few millimeters beyond the dorsal test when extended straight out laterally.
Formation and locality: Burgess shale, Middle Cambrian, on the west slope of the ridge between Mount Field and Wapta Peak, above Field, British Columbia.
The Appendages of Calymene and Ceraurus.
All of the work on these species has been done by Doctor Walcott, who summarized his results in 1881.
In the first of his papers (1875, p. 159), Walcott did not describe any appendages but paved the way for further work by a detailed and accurate description of the ventral surface of the dorsal shell of Ceraurus. He demonstrated the presence in this species of strongly buttressed processes which extend directly downward from the test just within the line of the dorsal furrows. One pair of these is seen beneath each pair of the glabellar furrows, each segment of the thorax has a pair, and there are four pairs on the pygidium. He pointed out also that these projections were but poorly developed on that part of the glabella which is covered by the hypostoma. He called them axial processes, the only name which appears to have been suggested thus far.
The first announcement of the discovery of actual appendages in Ceraurus and Calymene was made by the same investigator in a pamphlet published in 1876 in advance of the 28th Report of the New York State Museum of Natural History, the publication of the whole report being delayed till 1879. The results were obtained by the process of cutting translucent slices of enrolled trilobites derived from the Trenton limestone at Trenton Falls, New York. Since he summarized all the results of this study in one paper at a later date, it is not necessary to follow the stages of the work.
A second preliminary paper was published in pamphlet form in September, 1877, and in final form in 1879, when the first figures were presented.
In his important paper of 1881, Walcott reviewed all that was known of the appendages of trilobites to that time, and gave the results of seven years of study of sections of enrolled specimens. Slices had been made of 2,200 individuals from Trenton Falls, which resulted in obtaining 270 which were worthy of study. Of these, 205 were from Ceraurus pleurexanthemus, 49 from Calymene senaria, 11 from Isotelus gigas, and 5 from Acidaspis trentonensis.
Walcott's views on certain portions of the anatomy can best be set forth in the form of a few extracts (1881, pp. 199-208):
The Ventral Membrane.—In those longitudinal sections in which the ventral membrane is most perfectly preserved, it is shown to have been a thin, delicate pellicle or membrane, strengthened in each segment by a transverse arch, to which the appendages were attached. These arches appear as flat bands separated by a thin connecting membrane, somewhat as the arches in the ventral surface of some of the Macrouran Decapods….
In by far the greater number of sections, both transverse and longitudinal, the evidence of the former presence of an exterior membrane, protecting the contents of the visceral cavity, rests on the fact that the sections show a definite boundary line between the white calcspar, filling the space formerly occupied by the viscera, and the dark limestone matrix. Even the thickened arches are rarely seen.
The mode of attachment of the leg to the ventral surface is shown [in transverse and longitudinal sections of Ceraurus and Calymene]. These illustrations are considered as showing that the point of articulation was a small, round process projecting from the posterior surface of the large basal joint, and articulating in the ventral arch somewhat as the legs of some of the Isopods articulate with the arches in the ventral membrane. The arches of the ventral membrane in the trilobite … afford a correspondingly firm basis for the attachment of the legs.
Branchial appendages.—The branchiæ have required more time and labor to determine their true structure than any of the appendages yet discovered. They were first regarded as small tubes arranged side by side, like the teeth in a rake; then as setiferous appendages, and finally as elongate ribbon-like spirals and bands attached to the side of the thoracic cavity, the epipodite being a so-called branchial arm. All of these parts are now known to belong to the respiratory system, but from their somewhat complex structure, and the various curious forms assumed by the parts when broken up and distorted, it was a long time before their relations were determined.
The respiratory system is formed of two series of appendages, as found beneath the thorax. The first is a series of branchiæ attached to the basal joints of the legs, and the second, the branchial arms, or epipodites.
The branchiæ, as found in Calymene, Ceraurus, and Acidaspis, have three forms. In the first they bifurcate a short distance from the attachment to the basal joint of the leg, and extend outward and downward as two simple, slender tubes, or ribbon-like filaments. In the second form they bifurcate in the same mariner, but the two branches are spirals. These two forms occur in the same individual but, as a rule, the more simple ribbon-like branchia is found in the smaller or younger specimens, and the spiral form in the adult…. The spiral branchiæ of Ceraurus are usually larger and coarser than those of Calymene.
The third type of the branchiæ [consists of rather long straight ribbons arranged in a digitate manner on a broad basal joint]. As far as yet known, this is confined to the anterior segments of the thorax.
The epipodite or branchial arm was attached to the basal joints of the thoracic legs and formed of two or more joints. This has been called a branchial arm, not that it carried a branchia, but on account of its relation to the respiratory system. It is regarded as an arm or paddle, that, kept in constant motion, produced a current of water circulating among the branchiæ gathered close beneath the dorsal shell. . . .
Of the modification the respiratory apparatus underwent beneath the pygidium, we have no evidence.
In his latest publication (1918, pp. 147-153, pls. 26-28, 33), Walcott has reviewed his earlier work on Calymene and Ceraurus, and presented a new restoration of the former. The coxopodites are now interpreted as being similar to those of Triarthrus and Neolenus, but the exopodites are still held to be spiral and the setiferous organs labelled as epipodites rather than exopodites.
Comparison of the Appendages of Calymene and Ceraurus with those of Triarthrus.
As one may see by reading the above quotations from Doctor Walcott's descriptions, he found certain branchial organs in Ceraurus and Calymene which have not been found in other trilobites but otherwise the essential features of the appendages of all are in agreement.
It is now necessary to inquire if the thin sections can not be interpreted on the basis of trilobites with the same organs as Triarthrus. The interpretation of the structures seen in these translucent slices is exceedingly difficult, and Doctor Walcott deserves the utmost praise for the acumen with which he drew his deductions. Even with the present knowledge of Triarthrus, Isotelus, and Neolenus as a guide, I do not think it is safe to speak dogmatically about what one sees in them.
Walcott has summarized his results in his restoration of the appendages of Calymene (1918, pl. 33). The coxopodite supports a slender six-jointed endopodite as in Triarthrus, dorsal to which is a short setiferous epipodite which differs from the exopodite of Triarthrus, in being less long, unsegmented, and in having shorter setæ. Arising from the same part of the coxopodite with this epipodite is the bifurcate spiral branchia which has not been seen in this form in other trilobites. The evidence on which the existence of this organ is postulated consists of a series of sections across the thorax, the best of them figured by Walcott in his plates 2 and 3 (1881) and plate 27 (1918).
The specimens sliced were all partially or quite enrolled, and in that position one would expect to find the appendages so displaced that it would be only rarely that a section would be cut, either by chance or design, in such a direction as to show any considerable part of any one appendage. This expectation has proved true in regard to the endopodites, the sections rarely showing more than two or three consecutive segments. Sections like those shown in figures 1 and 2 in plate 2 (1881) seem to be unique. On the other hand, there are numerous slices showing the so-called spiral branchiæ. They show for the most part as a succession of rectangular to kidney-shaped spots of clear calcite.[1] Usually these clear spots are isolated, not confluent, but in a small number of specimens, perhaps three or four, the spots are connected in such a way as to show a zig-zag band which suggests a spiral. Such an explanation is of course entirely reasonable, but it would be surprising if so slender a spiral should be cut in such a way as to exhibit the large series of successive turns shown in many of these thin sections. Continuous sections of such organs should be no more common than continuous sections of endopodites.
[1] In looking at Walcott's figures of 1881, it should be remembered that the dark portions of the figures are clear calcite in the specimens, while the light part is the more or less opaque matrix.
One of the arguments against the interpretation of these series of spots as sections across spiral arms is that of probabilities. It is known from flattened specimens that Neolenus, Kootenia, Ptychoparia, Triarthrus, and Cryptolithus all have a single type of exopodite, consisting of a simple setiferous shaft. All these genera have been examined in a way that permits no doubt about the structure, and no trace of spiral arms has been detected. On the other hand, Walcott found spiral arms in three unrelated genera, Calymene, Ceraurus, and Acidaspis, all of the trilobites in which he found exopodites by the method of sectioning. What are the probabilities that genera of three different families, studied by means of sections, should agree in having a type of exopodite different from that of the five genera about whose interpretation there can be no doubt?
Another argument against the interpretation of the sections as spirals is that in any one line the individual spots are of roughly uniform size. This means of course that the spiral has been cut by a plane parallel to the tangent plane. This might happen once, just as once Doctor Walcott cut all six segments of a single endopodite, but that it should happen [49] repeatedly is highly improbable. Moreover, there is a limit to the diameter of the section which may be made from these slender spirals. Most of the spots have one diameter about one half greater than the other, but others are from three to six times as long as wide. These last could obviously be cut only from a very large spiral, and they are therefore interpreted by Walcott as setæ of epipodites. Yet all gradations are found among the sections, from the long setæ to the short dots. (See pl. 27, 1918.) In referring to one slice, Walcott says (1918, p. 152):
In the latter figure and in figure 13, plate 27, the setæ of several epipodites appear to have been cut across so as to give the effect of long rows of setæ. The same condition occurs in specimens of Marrella when the setæ of several exopodites are matted against each other.
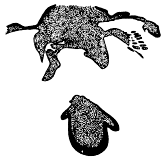
Fig. 12.—A slice of Ceraurus pleurexanthemus in which the exopodite happened to be cut in such a way as to show a part of the shaft and some of the setæ in longitudinal section. Specimen 80. × 4.
This is certainly an apt comparison, and equally true if Neolenus, Triarthrus, or Cryptolithus were substituted for Marrella.
Now consider the "epipodites." They are well shown in Calymene in the specimens illustrated on plate 27, figure 11 (1918), and plate 3, figure 3 (1881), and less clearly in one or two others. Slices 22 (pl. 27, fig. 12, 1918) and 80 (our fig. 12) show what is called the same organ in Ceraurus. It will be noted that all of these slices are cut in the same way, that is, more or less parallel to the under surface of the head, or, at any rate, on a plane parallel to a plane which would be tangent to the axial portion of the coiled shell. The sections which show the spirals best are those which are cut by a plane perpendicular to the long axis of the body. If one were to attempt to cut an enrolled Triarthrus in such a way as to get a section showing the length of the setæ, one would not cut a section perpendicular to the axis of the animal, nor, in fact, would he cut one parallel to the ventral plane, but it is obvious that in this latter type of section he would stand a better chance of finding a part of the plane of the exopodite coincident with the plane of his section than in the former. And that seems to be what has happened in these sections of Calymene and Ceraurus. If the exopodites were preserved, transverse sections were bound to cut across many sets of fringes, and the resultant slice would show transverse sections of the setæ as a series of overlapping spots. A few fortunately located sections in a more nearly horizontal [50] plane might cut the setæ and occasionally the shaft of one or more exopodites in the longitudinal plane, and the resulting effect would produce the so-called "epipodites." A careful study has shown that no one of these epipodites is complete, and they do not have the palmate form shown in Walcott's figures.
And the last and most important argument against the spiral appendages is that certain slices, of both Calymene and Ceraurus, show definitely exopodites of exactly the type found in other trilobites. These are discussed later in the detailed description of the various slices.
If these series of spots are interpreted on the basis of the known structure of Triarthrus, they are of course a series of sections through the setæ of the exopodites. It will be shown in Part IV that these setæ are not circular in section, but flattened, in Cryptolithus even blade-like, and that they overlap one another. A section across them would give the same general appearance as, for instance, that shown in figures 4, 6, 9, and 10 of Walcott's plate 3 (1881).
When both endopodites and the "spiral branchiæ" are present in the same section (pl. 1, fig. 4; pl. 2, figs. 1, 2), the "spiral branchiæ" are dorsal to the endopodites, as the setæ of the exopodites would be expected to be. The specimens which show the clear spots connected, and which suggest a spiral (pl. 3, fig. 5), may seem at first sight to bear evidence against this interpretation, but one has only to think of the effect of cutting a section along the edge where the setæ are attached to the shaft of the exopodite of Triarthrus to see that such a zig-zag effect is entirely possible. One would expect to cut just this position only rarely, and, in fact, the zig-zags are seen in only three or four sections. The bifurcation of the basal segment of the "spiral branchiæ" (pl. 3, fig. 10, 1881) is probably more apparent than real, if indeed these basal segments have anything to do with the succeeding one.
A second peculiarity of Calymene, shown in Walcott's restoration, is the great enlargement of the coxopodites and of the distal segments of the endopodites of the fifth pair of appendages of the cephalon. This is based on the sections of plate 3, figures 6, 7, 8, 9, 10 (1881). After a study of the specimens I regret to find myself still unconvinced that the posterior cephalic appendages were any larger than those in front.
The most striking value of the thin sections of Ceraurus and Calymene, and therein they have a great superiority over all the other forms so far investigated, is that they show the extent of the body cavity and the position, though not the substance, of the ventral membrane. Transverse sections through Ceraurus (Walcott's pl. 1. figs. 1-5; pl. 2, figs. 1, 3, 1881) and Calymene (pl. 3, figs. 9, 10, 1881) show that the body cavity was almost entirely confined to the axial lobe. The longitudinal sections of Ceraurus (pl. 2, figs. 6, 8; pl. 4, fig. 8) and of Calymene (pl. 2, figs. 5, 7; pl. 5, figs. 1-4) show that the ventral membrane was exceedingly thin and was wrinkled transversely when the shell was enrolled.
The specimens of figures 1-3, plate 5 (1881) show the form of the ventral membrane more distinctly than any of the others. The section of figure 1 was cut just inside the dorsal furrow on the right side, and figure 2, which is on the opposite side of the same slice, is almost exactly on the median line. Figure 3 shows a section just inside the left dorsal furrow. Section 2 did not cut any of the appendages, and the ventral membrane is [51] shown as a thickened, probably chitinous sheet thrown into low sharply crested folds equal in number to, and pointing in a direction just the reverse of, the crests of the segments of the thorax. Under the pygidium, where there would of course be less wrinkling, the folds are hardly noticeable. In the actual specimens one sees more plainly than in the figures the line of separation between the ventral membrane and the appendages, but the state of preservation of everything beneath the dorsal shell is so indefinite that one does not feel sure just what the connection between the appendages and the membrane was. In the original of figure 5, plate 2, which seems to have been cut so as to cross the appendages at their line of junction with the ventral membrane, there appear to be narrow chitinous (?) plates extending from the ventral membrane to the dorsal test.
In Ceraurus there are regular calcareous processes which extend down from the dorsal test just inside the line of the dorsal furrow, and which undoubtedly serve as points of attachment of the appendages. These processes, which for convenience I have designated as "appendifers," are broken off in most specimens showing the lower surface of Ceraurus pleurexanthemus, but on certain ones cleaned with potash they are well preserved. Doctor Walcott showed them well in his figures of the lower surface of this species (1875, pl. 11; 1881, pl. 4, fig. 5), while the attempt of Raymond and Barton (1913, pl. 2, fig. 7) to show them by photography was not so successful.
There is one pair of appendifers on each of the thoracic segments and four pairs on the pygidium. On the cephalon there is one pair under the neck furrow, and a pair under the posterior glabellar furrows. These are not concealed by the hypostoma. Further forward, and completely covered by the hypostoma, are two much less strongly developed but similar ones, so that there are in all four pairs of appendifers on the cephalon, though it is extremely doubtful if the appendages were articulated directly to all of them. On a specimen of Ceraurus pleurexanthemus 30 mm. long on the median line, the dorsal furrows are 7.5 mm. apart at the anterior end of the thorax, and the tips of the appendifers of this segment are only 4 mm. apart. Each consists of a straight slender rod with a knoblike end projecting directly downward from the dorsal test, and supported by a thin calcareous plate which runs diagonally forward to the anterior edge of the segment directly under the dorsal furrow. On the pygidium three pairs of the appendifers have this form, while the fourth pair consist of low rounded tubercles which are concealed by the doublure. These appendifers are probably cut in many of Walcott's sections of Ceraurus, but owing to the state of preservation it is not always possible to determine what part is appendage, what part is body cavity, and what part is appendifer.
Nearly forty years ago Von Koenen (1880, p. 431, pl. 8, figs. 9, 10) described and figured the appendifers of Phacops latifrons. He found them to be calcareous projections on the hinder margin of each segment, converging inward, and about 1.5 mm. long. He correctly considered them as supports (Stützpunkte) for the feet.
Appendifers are well developed also in Pliomerops, and in well preserved specimens of Calymene senaria from Trenton Falls they are present, but instead of being rod-like processes, they are rather thick, prominent folds of the shell. They are also well shown in some of the thin sections. A specimen of Triarthrus (No. 229, our pl. 5, fig. 2) has broad processes extending downward from the lower side of the test below the dorsal furrows, much as in Calymene, and the individual of Cryptolithus shown in plate 8, figure 1, [52] possesses slender appendifers. Two other specimens (Nos. 237 and 242) show them quite well. They were probably present in all trilobites, but seldom preserved. The appendifers have the same origin as the entopophyses of Limulus, and like them, may have relatively little effect on the dorsal surface.
Illustrated: Walcott, Bull. Mus. Comp. Zool., Harvard Coll., vol. 8, 1881, pl. 1, figs. 6-10; pl. 2, figs. 5-7, 10; pl. 3, figs. 1, 3, 8-10; pl. 4, figs. 3, 7; pl. 5, figs. 1-6; pl. 6, figs. 1 (restoration), 2;—Proc. Biol. Soc. Washington, vol. 9, 1894, pl. 1. fig. 7 (restoration);—Geol. Mag., dec. 4, vol. 1. 1894, pl. 8, figs. 7, 8;—Smithson. Misc. Coll., vol. 67, 1918, pl. 26, figs. 1-7, 9-13; pl. 27, figs. 4, 5 (not 5a), 11 (not 12, Ceraurus), 13, 14, 15 (not Ceraurus); pl. 28, figs. 7, 8; pl. 33, fig. 1 (restoration); pl. 34, fig. 2; pl. 35, fig. 6.—Dames, N. Jahrb. f. Min., etc., vol. 1, 1880, pl. 8, figs. 1-5.—Milne-Edwards, Ann. Sci. Nat., Zoologie, ser. 6, vol. 12, 1881, pl. 11, figs. 19-32; pl. 12, figs. 33-41.—Packard, Amer. Nat., vol. 16, 1882, p. 796, fig. 12.—Bernard, The Apodidæ, 1892, text figs. 50, 52, 54;—Quart. Jour. Geol. Soc., London, vol. 50, 1894, text figs. 13, 15, 17.—Œhlert, Bull. Soc. Géol. France, ser. 3, vol. 24, 1896, fig. 12.—Beecher, Amer. Jour. Sci., vol. 13, 1902, pl. 5, fig. 7.
In both of Walcott's accounts (1881, 1918) of the appendages of Calymene and Ceraurus, he has described them together, so that those who have not taken time to study the illustrations and disentangle the descriptions are very apt to have a confused notion in regard to them. I have therefore selected from the original specimens those slices of Calymene which are most instructive, and bearing in mind the probable appearance of the appendages of an enrolled Triarthrus, have tried to interpret them. In such a method of study, I have of course started with a pre-formed theory of what to expect, but have tried to look for differences as well as likenesses.
Antennules.—The evidence of antennules rests on a single slice (No. 78). The appendage in question is exceedingly slender and arises at the side of the hypostoma near its posterior end. It shows fine, slender segments, and curves first outward and then forward. If it is in its natural position, it is not an antennule, but the endopodite of the second or third pair of cephalic appendages. It is short, only about one-third the length of the hypostoma, but is doubtless incomplete. The two distal segments show a darker filling, indicating that they were hollow. Judging from analogy with other trilobites, the appendage is probably an endopodite and not an antennule. There can be no reasonable doubt, however, that Calymene possessed antennules.
Some idea of the form of the coxopodites of the cephalic appendages may be obtained from sections which cut in approximately the plane of the hypostoma. Such sections are shown in Walcott's photographs (pl. 26, figs. 4, 6, 11, 1918). Specimens 50 (fig. 4, our fig. 13), 51 (fig. 6), 6 (fig. 11), and 40 (our fig. 14) agree in showing two pairs of slender coxopodites which are attached at the sides of the hypostoma and run backward parallel and close to it, and two pairs of larger coxopodites which are behind the hypostoma, although the point of attachment of the third pair is in front of its tip. The anterior pair are apparently under-developed and no longer function as mouth parts, while the posterior two pairs are large and armed on their inner ends with spines. Specimen 78, which has already been mentioned in connection with the antennules, shows a second very slender appendage back of the so called antennule, which is equally slender, but is directed outward instead of forward. [53] It seems not improbable, from their position and similarity, that these two are the endopodites of the first two appendages on one side of the hypostoma. Specimen 6 shows rather inadequately the endopodites of the second and third cephalic appendages. I have not found other slices showing endopodites of the cephalon. Walcott, in both his restorations, has shown enlarged, paddle-shaped dactylopodites on the distal ends of the fourth cephalic endopodites. The evidence for this rests principally on three slices, No. 38 (pl. 26, figs. 9, 10), 53 (pl. 26, fig. 12), and 43 (pl. 26, fig. 13). Of these, No. 43 may be dismissed at once as too poorly preserved to be interpreted. No. 53 does show a section of an appendage which seems to have an unusually wide dactylopodite, but this slice presents no evidence at all as to the appendage to which the dactylopodite appertains, nor can one even be sure that there has not been a secondary enlargement. Specimen 43 shows this feature much less definitely than is indicated by the published photograph and drawing. The segment in question is strongly curved, with a constriction possibly dividing it into two. If it is in its natural position in this section, it obviously belongs to one of the thoracic segments and not to the cephalon. With evidence of difference so unsatisfactory, I prefer to reconstruct the posterior cephalic endopodites on the same plan as those of the thorax.
Exopodites.—Walcott admits that there is no direct evidence of spiral exopodites in the cephalon of Calymene. No one of the sections cutting through the plane of the hypostoma shows any trace of appendages which could be interpreted as exopodites.
The large coxopodites of the anterior thoracic appendages are well shown in many specimens cut longitudinally, of which Nos. 23, 50, and 55 may be mentioned, since photographs of them have been published by Walcott (pl. 26, figs. 1-4, 1918). The endobases of all taper toward the proximal ends. Transverse slices show sections of the coxopodites which [54] are no wider than those in longitudinal sections, indicating that they were not compressed but probably cylindrical. This is borne out by an individual (pl. 28, fig. 7, 1918) which is not a slice but an actual specimen, the body cavity of which was hollow, and, opened from above, shows the impressions of the last two coxopodites of the cephalon, and the first four of the thorax.
One transverse section (No. 63, see our fig. 15) is especially valuable, as it shows the method of articulation of the coxopodites with the dorsal skeleton. Another specimen (No. 73) shows that appendifers are present in Calymene, and while the appendifer does not retain its original form in slice No. 63, the section does show clearly that there was a notch in the inner (upper) side of the coxopodite into which the lower end of the appendifer fitted, thus giving a firm, articulated support for the appendage. This notch appears to be slightly nearer the outer than the inner end of the coxopodite, and since it must have made a kind of ball-and-socket joint, considerable freedom of movement was allowed. The appendage must have been held in place by muscles within the coxopodite and attached to the appendifer.
No slice which I have seen shows a continuous section through all the segments of an endopodite, but many, both longitudinal and transverse, show one, two, or as many as three segments.
Such sections as No. 120 show that the endopodites of the thorax were slender and composed of segments of rather uniform diameter. Other sections, notably No. 83, 154, and in, show that they tapered distally, and bore small spines at the outer end of each segment.
The exopodites of course furnish the chief difficulty in interpretation. Doctor Walcott finds two sets of structures attached to the coxopodite, a long, slender, spiral exopodite, and a short, broad epipodite with a fringe of long setæ. Since he has given the same interpretation for Calymene, Ceraurus, and Acidaspis, I have considered the question of all three together on a preceding page (p. 48), and given my reasons for regarding both structures as due to sections in different directions across setiferous exopodites.
Sections like those shown in figures 11, 13, and 14 of plate 27 (1918) happen to be cut in or near the plane of the setæ of an exopodite, and so show hairs of considerable length. Such sections are, as would be expected, very few in number, while sections like those shown on figures 4, 5, 7, and 9 of plate 27, which cut the setæ more nearly at right angles, are very common. Slices which give any definite idea of the form of the shaft of the exopodite are exceedingly rare. Perhaps the most satisfactory one is No. 23 (pl. 3, fig. 3, 1881), which shows the proximal part of a long, slender, unsegmented shaft, with the bases of a number of slender setæ. The organ is not complete, as would be inferred from the published figure, but the section cuts diagonally across it, and the total length is unknown. It is directed forward, like the exopodites of Neolenus, but whether or not this is a natural position is yet to be learned.
The proximal, non-setiferous portion of the exopodite is evidently at an angle with the setiferous part. Another similar exopodite is apparently shown by specimen 29 (pl. 3, fig. 9, 1881), which has a similar angulated shaft and just a trace of the bases of the setæ.
That appendages were present under the pygidium is shown by longitudinal sections, but nothing is known of the detail of structure.
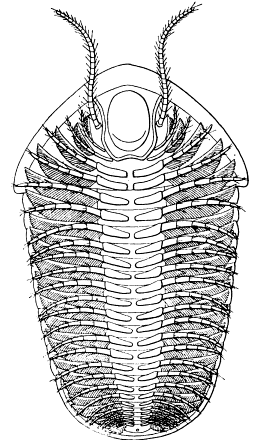
Fig. 16. Restoration of Calymene senaria Conrad, based upon data obtained from the study of the translucent sections made by Doctor Walcott. Prepared by Doctor Elvira Wood, under the supervision of the author. About twice natural size.
Relation of Hypostoma to Cephalon in Calymene.
In Calymene the shape of the hypostoma bears little relation to the shape of the glabella, and it is relatively smaller, both shorter and narrower, than in Ceraurus. In shape, neglecting the side lappets at the front, it is somewhat rectangular, but rounded at the back, where it is bifurcated by a shallow notch. The anterior edge has a narrow flange all across, which is turned at almost right angles to the plane of the appendage, and which fits against the doublure of the free cheeks at the sides and against the epistoma in the middle. The side lappets show on their inner (upper) surface shallow pits, one on each lappet, which fit over projections that on the dorsal surface show as deep pits in the bottom of the dorsal furrows in front of the anterior glabellar furrows. The appendifers on the head in Calymene take the form of curving projections of shell underneath the glabellar and neck furrows, and owing to the narrowness of the hypostoma, all these are visible from the ventral side, even with it in position. This shield extends back about 0.6 of [56] the length of the cephalon, and to a point a little behind the second glabellar furrow from the back of the head.
In Doctor Walcott's restoration of Calymene he has represented all four pairs of biramous appendages as articulating back of the posterior end of the hypostoma. I think his sections indicate that the gnathobases of two pairs of these appendages rested alongside or beneath it, and in particular, the longitudinal sections (1881, pl. 5) would appear to show that the mouth was some distance in advance of its posterior end.
(Text fig. 16.)
From what has been said above, it is evident that for a restoration of the appendages of Calymene considerable dependence must be placed upon analogy with other trilobites. Nothing is positively known of the antennules, the exopodites of the cephalon, or any appendages, other than coxopodites, of the pygidium, but all were probably present. It is inferred from the slices that the first two pairs of cephalic appendages were poorly developed, the endopodites short and very slender, the coxopodites lying parallel to the sides of the hypostoma and nearly or quite functionless. The gnathites of the last two pairs of cephalic appendages are large, closely approximated at their inner ends, and bear small tooth-like spines. The endopodites are probably somewhat better developed than the anterior ones and more like those on the thorax.
The coxopodites of the thorax appear to have had nearly cylindrical endobases which tapered inward. The endopodites were slender, tapering gradually outward, and probably did not extend beyond the dorsal test. Small spines were present on the distal end of each segment. Each exopodite had a long, slender, unsegmented shaft, to which were attached numerous long, overlapping, flattened setæ. The shaft may have been angulated near the proximal end, and may have been directed somewhat forward and outward as in Neolenus, but the evidence on this point is unsatisfactory. The number of pairs of appendages is that determined by Walcott from longitudinal sections, namely, four pairs on the cephalon beside the antennules, thirteen pairs in the thorax, and nine pairs on the pygidium.
Illustrated: Walcott, Bull. Mus. Comp. Zool., Harvard Coll., vol. 8, 1881, pl. 6, figs. 5a, b;—Proc. Biol. Soc. Washington, vol. 9, 1894, pl. 1, fig. 10;—Geol. Mag., dec. 4, vol. 1, 1894, pl. 8, fig. 10;—Smithson. Misc. Coll., vol. 67, 1918, pl. 36, figs. 1, 2, 2a-d.—Milne-Edwards, Ann. Sci. Nat., Zoologie, ser. 6, vol. 12, 1881; pl. 12, figs. 44a, b.
In the United States National Museum there is a thin piece of limestone, about 3 inches square, which has on its surface eight jointed objects that have been called legs of trilobites. Two of these were figured by Walcott (1881, pl. 6, fig. 5). The slab contains specimens of Dalmanella and Cryptolithus, in addition to the appendages of trilobites, and is said by Doctor Ulrich to have come from the tipper part of the Point Pleasant formation (Trenton) on the bank of the Ohio River below Covington, Kentucky.
The specimens are all endopodites of long slender form, similar to those of Triarthrus, but since that genus does not occur in the Point Pleasant, it is necessary to look upon some other trilobite as the former possessor of these organs. Both Isotelus and Calymene occur [57] at this horizon, and as the specimens obviously do not belong to Isotelus or Cryptolithus, it is probable that they were formerly part of a Calymene.
All the endopodites are of chitinous material, and the various specimens show, according to the perfection of their preservation, from four to six segments. The endopodite as a whole tapers but slightly outward, and the individual segments are of nearly equal length. They appear to be but little crushed, and are oval in section, with a crimped anterior and posterior margin. One or two show a median longitudinal ridge, such as is seen in some appendages of Triarthrus. Each segment is parallel-sided, with a slight expansion at the distal end, where the next segment fits into it.
Under the heading "Ordovician Crustacean Leg," Walcott (1918, p. 154, pl. 36, figs. 1,2) has recently redescribed these specimens, and thinks that they do not belong to Calymene, nor, indeed, to any trilobite. He concludes that they were more like what one would expect in an isopod. Passing over the fact that the oldest isopod now known is Devonian, the fossils in question seem to me quite trilobite-like. Walcott says:
The legs are associated with fragments of Calymene meeki but it is not probable that they belong to that species; if they did, they are unlike any trilobite leg known to me. The very short coxopodite and basopodite are unknown in the trilobites of which we have the legs, as they are fused into one joint forming the long protopodite in the trilobite. The distal joint is also unlike that of the trilobite legs known to us.
A great deal of Doctor Walcott's difficulty probably arises from his homology of the coxopodite of the trilobite with the protopodite of the higher Crustacea. The coxopodite of the trilobite is not fused with the basipodite, this latter segment always remaining free. Indeed, Walcott himself says of Neolenus (1918, p. 128):
Each thoracic leg (endopodite) is formed of a large elongate proximal joint (protopodite), four strong joints each about 1.5 times as long as wide (basopodite, ischiopodite, meropodite and carpopodite); two slender elongate joints (propodite and dactylopodite) and a claw-like, more or less tripartite termination.
Walcott's drawing (pl. 36, fig. 1) is a composite one, and while it shows eight segments, I was not able to count more than seven on any of the specimens themselves. In regard to the terminal segment, the dactylopodite of the limb shown in his plate 36, figure 2, is unusually long, and a comparison with other photographs published on the same plate shows that such long segments are unusual.
Proof that these are appendages of a Calymene is of course wanting, but there is no particular reason so far to say that they are not.
Measurements: Two of the more complete specimens, each showing six segments, are each 8 mm. long.
Somewhat similar to the specimens from Covington are the ones described by Eichwald (1825, p. 39, 1860, pl. 21), the specimens being from the Silurian of Gotland. The figure copied by Walcott (1881, pl. 6, fig. 4) has never been looked upon as entirely satisfactory evidence of the nature of the specimen, and so far as I know, the fossil has not been seen by any modern investigator.
Ceraurus pleurexanthemus Green.
(pl. 11; text figs. 12, 17-19, 21, 22, 24, 29, 30.)
Illustrated: Walcott, Ann. Lye. Nat. Hist. New York, vol. II, 1875, pl. 11;—31st Ann. Rept. New York State Mus. Nat. Hist, 1879, pl. 1, fig. 3;—Bull. Mus. Comp. Zool., Harvard Coll., vol. 8, 1881, pl. 1, figs. 1-5; pl. 2, figs. 1-4, 6-8; pl. 3, figs. 2, 4-7; pl. 4, figs. 1, 2, 4-6, 8; pl. 6, fig. 3; Smithson. Misc. Coll., vol. 67, [58] 1918, pl. 26, figs. 8, 14, 15; pl. 27, figs. 1-3, 5a, 6-9, 12 (not Calymene), (not 15, Calymene); pl. 28, figs. 1-5; pl. 34, fig. 1; pl. 35, fig. 7.—Milne-Edwards, Ann. Sci. Nat., Zoologie, ser. 6, vol. 12, 1881, pl. 10, figs. 1-18.—Bernard, The Apodidæ, 1892, text figs. 46, 51.
No trace of antennules has yet been found.
I find only three sections cut through the plane of the hypostoma of Ceraurus which show anything of the cephalic appendages, and no one of them is very satisfactory. The best is No. 22, the one figured by Walcott (pl. 3, fig. 2, 1881; pl. 27, fig. 12, 1918), but one should remember that this section is not actually cut in the plane of the hypostoma but is a slice diagonally through the head, cutting through one eye and the posterior end of the hypostoma. It shows what seem to be the coxopodites of the second, third, and fourth pairs of cephalic appendages, the exopodites of the third and fourth pairs, and the metastoma. If this interpretation is correct, the first pair of gnathites lay alongside the hypostoma or under its edge, and were feebly developed, the second pair were attached in front of the tip of the hypostoma, curved back close to it, and their inner ends reached the sides of the metastoma. The third and fourth pairs were back of the metastoma, the third pair was stronger than the second, and the fourth probably like the third.
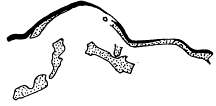
Fig. 17. Transverse section of Ceraurus pleurexanthemus, showing the relation of the coxopodite to the appendifer. Traced from a photographic enlargement of the slice. Specimen 128. × 4/5.
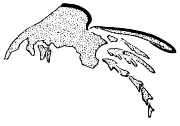
Fig. 18. Slice of Ceraurus pleurexanthemus, showing a nearly continuous section of an endopodite and an exopodite above it. The latter is so cut as to show only the edge of the shaft and the bases of a few setæ. Traced from a photographic enlargement. Specimen in. × 4.
Specimen 92 shows traces of the slender endopodites belonging to the cephalon, but no details. Specimen 22 shows on one side exopodites (epipodites of Walcott) belonging to the third and fourth cephalic appendages. That belonging to the third shows some long setæ and a trace of the shaft, while the one on the fourth appendage (third coxopodite) has a portion of a broad shaft and a number of long setæ. It should again be remembered that the slice does not cut through the plane of the exopodite, but across it at a low angle, so that a part but not all of the shaft is shown. On the other side of this slice there is a fairly good section of one of the thoracic exopodites. It is, however, turned around in the opposite direction from the others, as would be expected in an enrolled specimen.
Specimens 4 and 5 (pl. 1, figs. 4, 5, 1881) are slices cut diagonally through the head of Ceraurus, in front of the posterior tip of the hypostoma. They show fragments of endopodites and exopodites which may be interpreted as practically identical in form with those of the thorax. Due to the diagonal plane in which the section is cut, slice 5 shows [59] the coxopodites of two pairs of appendages, one lying nearer the median cavity than the other. It is extremely difficult to visualize the interpretation of such sections.
A transverse section through a thoracic segment (No. 128, our fig. 17) shows the relation of coxopodite to appendifer to be the same as in Calymene, the upper side of the coxopodite having a notch a little outward from the middle. After seeing that specimen, it is possible to understand slice No. 168, which shows longitudinal sections through a number of coxopodites of the thorax, with fragments of both exopodites and endopodites articulated at the distal ends. These and longitudinal vertical sections like No. 18 (pl. 2, fig. 8, 1881) show that the endobases taper inward, and the general uniformity in width in sections taken at various angles indicates that the coxopodites were not greatly flattened.
A unique slice (No. 111, pl. 2, fig. 2, 1881; pl. 27, fig. 1, 1918; our fig. 18) shows a nearly complete thoracic endopodite, and above it a part of the proximal end of the exopodite of the same segment. When one considers that out of over two thousand sections only this one shows the six successive segments of an endopodite, one realizes how futile it is to expect that dozens of the equally slender "spirals" should be cut so as to show practically all their turns.
This endopodite is slender, all the segments have nearly the same length and diameter, though there is a slight taper outward, each segment is expanded distally for the articulation of the next, and there are small spines on the distal ends of some of them. There is probably a terminal spine present, though it is neither so long nor so plainly visible as in Walcott's photograph.
The exopodite on this same specimen was evidently cut diagonally across near the setiferous edge, showing a section through the shaft and the bases of seven setæ (fig. 18). This section is so exactly what would be obtained by cutting similarly an exopodite of either Neolenus or Triarthrus that it should in itself dispose of the "spiral-exopodite" theory.
Several sections have already been illustrated showing sections across the setæ of the exopodites (pl. 3, figs. 4-6, 1881; pl. 27, figs. 3, 4, 9, 1918), and similar sections are not uncommon. Only a very few, however, show sections in the plane of the exopodite. If only No. 111, described above, were known, it would be inferred that the exopodite had a slender shaft as in Calymene, but another good slice, No. 80 (fig. 12, ante) shows that the blade was rather broad, though not so broad as in Neolenus. The other specimen is No. 22, which has already been discussed. The thoracic exopodite of this specimen has been very incorrectly figured by Walcott, as it shows no such palmate shaft as he has indicated, but a long blade-like one is outlined, though its entire width is not actually shown.
Sections 14 and 18 (pl. 2, figs. 4, 8, 1881) prove the presence under the pygidium of three pairs of appendages, the coxopodites and fragments of endopodites of which are shown. Nothing is known of the exopodites.
Relation of Hypostoma to Cephalon.
In Ceraurus the body portion and posterior end of the hypostoma are roughly oval, about as wide as the glabella at its broadest part, and the posterior edge extends back to [60] within 0.5 to 1 mm. of the neck furrow. The posterior pair of appendifers are behind the hypostoma, while the second pair are in front of its posterior end but escape being covered by it on account of its oval shape. At the anterior end the hypostoma is widened by the presence of two side lappets which extend beyond the boundaries of the glabella. In both Ceraurus and Cheirurus the anterior edge of the hypostoma fits against the doublure at the anterior margin of the head and the epistoma is either entirely absent or is so narrow as not to be seen in specimens in the ordinary state of preservation. A section across the cephalon of Ceraurus pleurexanthemus at the horizon of the eyes shows the sides of the hypostoma fitting closely against the sides of the glabella (Walcott's pl. 1, fig. 1). Further back on the head it is not in contact with the dorsal test, and the gnathobases extend beneath it.
Restoration of Ceraurus pleurexanthemus.
The restoration of the appendages of Ceraurus pleurexanthemus is a tentative one, based upon a careful study of the translucent sections prepared by Doctor Walcott. In no case among these sections is the actual test of any appendage preserved, and the real form of each part is generally obscured by the crystallization of the calcite which fills the spaces formerly occupied by animal matter.
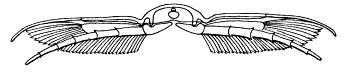
Fig. 19. Restoration of a transverse section of the thorax of Ceraurus pleurexanthemus Green, showing the relation of the appendages to the appendifers and the ventral membrane. The probable positions of the heart and alimentary canal are indicated.
No section shows anything which can be identified as any part of the antennules, so that these organs have been supplied from analogy with Triarthrus.
There are undoubtedly four pairs of biramous Cephalic appendages, but their points of attachment are not so obvious. There are two pairs of conspicuous appendifers on the posterior part of the cephalon and another pair almost concealed by the hypostoma. It is probable that the appendages of the cephalon were not attached directly beneath them, as the four pairs have to be placed within the space occupied by the three pairs of appendifers. As the mouth is in front of the posterior end of the hypostoma, the gnathites of the first pair of biramous appendages may have extended beneath that organ, or they may have lain beside it, and only become functional when the hypostoma was dropped down in the feeding position. The second pair of gnathites reached just to the tip of the hypostoma, and the other two pairs seemingly curved backward behind it.
The points of attachment on the thorax, as shown clearly in sections, were directly beneath the lower ends of the appendifers. The endopodites were long enough to reach to or a little beyond the outer extremities of the pleural spines, while the exopodites were apparently somewhat shorter. Each endopodite consisted of six short, fairly stout segments, each with at least two spines on the somewhat expanded distal ends. The exact [61] form of the exopodites could not be made out. The shaft was apparently rather short, unsegmented, and fairly broad. The setæ appear from the sections to have been more or less blade-shaped and to have overlapped, as do those of the exopodites of Cryptolithus. Judging from their position in the sections, the setæ not only bordered the posterior side of the shaft, but radiated out from the end as well.
The pygidium shows three pairs of functional appendifers, hence three pairs of appendages have been supplied. There is a fourth pair of rudimentary appendifers, but as they are beneath the doublure they could not have borne ambulatory appendages.
The Appendages of Acidaspis trentonensis Walcott.
A single individual of Acidaspis trentonensis, obtained from the same locality and horizon as the specimens of Triarthrus and Cryptolithus, when cleaned from the ventral side shows a number of poorly preserved endopodites which seem very similar in shape and position to those of Triarthrus. One endopodite on the right side of the head and the first five on the right side of the thorax are the best shown. All are slender, are directed first forward at an angle of about 45 with the axis, then, except in the case of the cephalic appendage, turn backward on a gentle curve and extend a little distance beyond the margin of the test, but not as far as the tips of the lateral spines of the thoracic segments.
The individual segments of the endopodites can not be seen clearly enough to make any measurements. On the fourth and fifth endopodites of the thorax, some of the segments seem to be broad and triangular as in Triarthrus. All that can be seen indicates that Acidaspis had appendages entirely similar to those of Triarthrus, but perhaps not quite so long, as they seem not to have projected beyond the limits of the lateral spines. There are no traces of antennules nor, unfortunately, of exopodites.
Measurements: Length 8 mm.
Walcott (1881, p. 206) stated that his sections had shown the presence in this species of legs "both cephalic and thoracic" and also the "spiral branchiæ." His specimens were from the Trenton at Trenton Falls, New York.
The Appendages of Cryptolithus.
Cryptolithus tessellatus Green.
(pl. 6, fig. 7; pls. 7-9; text figs. 20, 25, 45, 46.)
(See also Part IV.)
Illustrated: Beecher, Amer. Jour. Sci., vol. 49, 1895, pl. 3.
When Professor Beecher wrote his short article on the "Structure and Appendages of Trinucleus" (1895), he had only three specimens showing appendages. In his later work he cleaned several more, so that there are now thirteen specimens of Trinucleus = Cryptolithus available for study, though some of these do not show much detail. In his last and unpublished study, Beecher devoted the major part of his attention to this genus, and summarized his findings in the drawings which he himself made of the best individuals (text figs. 45, 46). Valiant (1901) stated that he had found a Trinucleus with antennæ in the Frankfort shale south of Rome, New York. The specimen has not been figured.
None of the specimens shows much more of the appendages of the cephalon than, the hypostoma and the antennules, so that we are still in ignorance about the mouth parts.
The most striking characteristics of the appendages are as follows: the antennules are long, and turn backward instead of forward; none of the limbs projects beyond the margin of the dorsal test; the exopodites extend beyond the endopodites, reaching very nearly to the margin of the test; the endopodites are not stretched out at right angles to the axis, but the first three segments have a forward and outward direction as in Triarthrus, while the last four turn back abruptly so that they are parallel to the axis; the limbs at the anterior end of the thorax are much more powerful than the others; the dactylopodites of the endopodites show a fringe of setæ instead of three spines as in Triarthrus and Neolenus. All these would, as Beecher has already suggested, seem to be adaptations to a burrowing habit of life, the antennules being turned backward and the other appendages kept within the shelter of the dorsal test in order to protect them, and the anterior endopodites enlarged and equipped with extra spines to make them more efficient digging and pushing organs.
(Text fig. 20.)
It should be definitely understood that the present figure is a restoration and not a drawing of a specimen, and that there are many points in the morphology of Cryptolithus about which no information is available, especially about the appendages under the central portion of the cephalon. The information afforded by all the figures published in this memoir is combined here. As gnathites are preserved on none of the specimens, those represented in the figure are purely conventional.
A person who is acquainted only with Cryptolithus preserved in shale, or with figures, usually has a very erroneous idea of the fringe It is not a flat border spread out around the front of the head, but stands at an angle about 45 in uncrushed specimens of most species. When viewed from the lower side, there is a single outer, concentric row of the cup-shaped depressions, bounded within by a prominent girder. This row is in an approximately horizontal plane, while the remainder of the doublure of the fringe rises steeply into the hollow of the cephalon. Since the front of the hypostoma is attached to this doublure, it stands high up within the vault and under the glabella. Two specimens, Nos. 231 and 233, show something of the hypostoma, and they are the only ones known of any American trinucleid. That of specimen 233, the better preserved, is very small, straight across the front, and oval behind. It seems that it is abnormally small in this specimen and I should not be surprised if in other specimens it should be found to be larger.
In the Bohemian Trinucleoides reussi (Barrande), the oldest of the trinucleids, the hypostoma is very commonly present, and is of the proper size to just cover the cavity of the glabella, seen from the lower side, and has, toward the anterior end, side flaps which reach out under the prominent glabellar lobes. This large size of the hypostoma would cause the antennules to be attached outside the dorsal furrows, and the position in which they are attached in the American species of Cryptolithus may be explained as an inherited one, since with the small hypostoma they might have been within the glabella, as in Triarthrus.
The antennules are seen in three specimens, and in all cases are directed backward. The particular course in which they are drawn in the restoration is purely arbitrary. The second pair of cephalic appendages are represented as directed downward and forward, since [63] in one or two specimens fragments of forward-pointing endopodites were seen near the front of the cephalon, and because in other trilobites the second pair of appendages is always directed forward. The remaining three pairs have a more solid basis in observed fact, for the two or three specimens retaining fragmentary remains of them indicate that they turn backward like those on the thorax, and that the individual segments are longer and more nearly parallel-sided than those of the more posterior appendages. The gnathites of all the cephalic appendages are admittedly purely hypothetical. None of the specimens shows them. As drawn, they are singularly inefficient as jaws, but if, as is suggested by the casts of the intestines of trinucleids found in Bohemia, these trilobites were mud-feeders, inefficient mouth-parts would be quite in order.
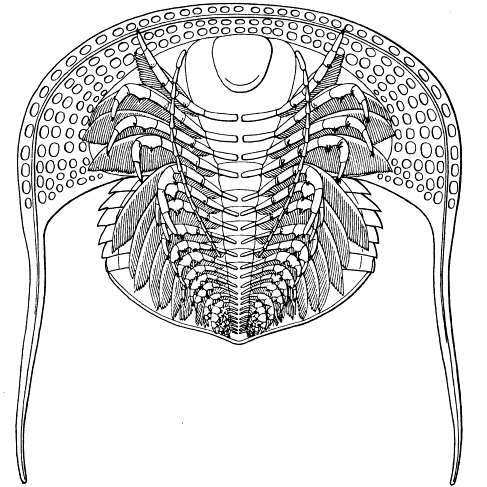
Fig. 20. Cryptolithus tessellatus Green. A restoration of the appendages drawn by Doctor Elvira Wood from the original specimens and from the photographs made by Professor Beecher. × 9.
The appendages of the thorax and pygidium can fortunately be taken quite directly from the photographs of the dorsal and ventral sides of well preserved specimens. There [64] is of course a question as to the number and the exact form of those on the pygidium, but I think the present restoration is fairly well justified by the specimens. As would be expected from the narrow axial lobe, the gnathobases of the coxopodites are short and small.
Summary on the Ventral Anatomy of Trilobites.
COMPARISON OF APPENDAGES OF DIFFERENT GENERA.
Since the appendages of Triarthrus, Cryptolithus, Neolenus, Calymene, and Ceraurus are now known with some degree of completeness, those of Isotelus somewhat less fully, and something at least of those of Ptychoparia, Kootenia, and Acidaspis, these forms being representatives of all three orders and of seven different families of trilobites, it is of some interest to compare the homologous organs of each.
All in which the various appendages are preserved prove to have a pair of antennules, four pairs of biramous limbs on the cephalon, as many pairs of biramous limbs as there are segments in the thorax, and a variable number of pairs on the pygidium, with, in the case of Neolenus alone, a pair of tactile organs at the posterior end. Each limb, whether of cephalon, thorax, or pygidium, consists of a coxopodite, which is attached on its dorsal side to the ventral integument and supported by an appendifer, an exopodite, and an endopodite. The exopodite is setiferous, and the shaft is of variable form, consisting of one, two, or numerous segments. The endopodite always has six segments, the distal one armed with short movable spines.
The coxopodite does not correspond to the protopodite of higher Crustacea, the basipodite remaining as a separate entity. The inner end of the coxopodite is prolonged into a flattened or cylindrical process, which on the cephalon is more or less modified to assist in feeding, and so becomes a gnathobase or gnathite. The inner ends of the coxopodites of the thorax and pygidium are also prolonged in a similar fashion, but are generally somewhat less modified. These organs also undoubtedly assisted in carrying food forward to the mouth, but since they probably had other functions as well, I prefer to give them the more non-committal name of endobases.
In Triarthrus and Neolenus the endobases are flattened and taper somewhat toward the inward end. In Isotelus, Calymene and Ceraurus, they appear to have been cylindrical. In other genera they are not yet well known. In all cases, particularly about the mouth, they appear to have been directed somewhat backward from the point of attachment. As it is supposed that these organs moved freely forward and backward, the position in which they occur in the best preserved fossils should indicate something of their natural position when muscles were relaxed.
Antennules.—Antennules are known in Triarthrus, Cryptolithus, Neolenus, and Ptychoparia. In all they are long, slender, and composed of numerous segments, which are spiniferous in Neolenus, and very probably so in the other genera.
In Triarthrus, Neolenus, and Ptychoparia they project ahead of the cephalon, emerging quite close together under the front of the glabella, one on either side of the median line. In Cryptolithus they turn backward beneath the body, but since only three or four specimens are known which retain them, it is possible that other specimens would show [65] that these organs were capable of being turned forward as well as backward. The proximal ends of the antennules being ball-like, it is probable, as Doctor Faxon has suggested to me, that these "feelers" had considerable freedom of motion. The antennules of Triarthrus are apparently somewhat less flexible than those of the other genera, and have a double curvature that is seen among the others only in Ptychoparia. The proximal end of an antennule in Triarthrus is a short cylindrical shaft, apparently articulating in a sort of ball-and-socket joint. The proximal end in the other genera is still unknown. The points of attachment in Triarthrus seem to be under the inner part of the second pair of glabellar furrows. In Cryptolithus they appear to be beside the anterior lobe of the glabella under what have long been known as the antennal pits. In the other genera the location is not definitely known, but in Neolenus it seems to be under the dorsal furrows near the anterior end of the glabella. Viewed from the under side, the point of attachment is probably always beside the middle or anterior part of the hypostoma, just behind the side wings.
Paired biramous appendages.—Behind the antennules all the appendages except those on the anal segment are biramous, consisting of a coxopodite with an inward-directed endobase and an outward-directed pair of branches, the exopodite above, and the six-jointed endopodite beneath. The basipodite really bears the exopodite, but the latter also touches the coxopodite. This structure has been seen in Triarthrus, Cryptolithus, Neolenus, Kootenia, Calymene, Ceraurus, and Ptychoparia. In Triarthrus, Neolenus, Acidaspis, Ptyclioparia, and Kootenia, the appendages extend beyond the margins of the dorsal test. In Cryptolithus and Isotelus none (other than antennules) does so. In Isotelus and Acidaspis only the endopodites have been seen. In Triarthrus, Calymene, Ceraurus, and Neolenus there are four pairs of appendages behind the antennules. The other genera probably had the same number, but the full structure of the under part of their cephala is not known. In Triarthrus the endopodites of the cephalon are slender, the individual segments parallel-sided, the inner ones flattened, the outer ones cylindrical in section. They project slightly beyond the edge of the cephalon when fully extended, and each terminates in three small spines. In Cryptolithus the endopodites of the cephalon are longer than those of the thorax, but with the possible exception of the first pair, are bent backward at the carpopodite, and do not ordinarily project beyond the brim of the test. In Neolenus the endopodites of the cephalon are rather thick and wide, but are long, project forward, and extend beyond the brim. The individual segments are flattened, probably compressed oval in section. The terminal segment of each is furnished with three strong spines at its distal end. In Calymene and Ceraurus the endopodites appear to consist of slender segments which are oval or circular in section. In Calymene Walcott believed the three distal segments of the last endopodites of the head to be greatly enlarged, giving these appendages a paddle-like form similar to some of the appendages of eurypterids. The evidence for this does not seem to me to be good. The cephalic endopodites of Isotelus are entirely similar to those of the thorax, and are rather short, consisting of a series of short cylindrical segments which do not taper greatly toward the distal end. The endopodites of the cephalon of Acidaspis, Kootenia, and Ptychoparia are still unknown.
The exopodites of the cephalon seem in all known cases (Triarthrus, Cryptolithus, Neolenus, and Ceraurus) to be like those of the thorax. They point more directly forward in most cases, project beyond the margin of the head normally only in Triarthrus, and usually occupy the region under the cheeks (fixed and free).
The endobases of the coxopodites of the appendages of the cephalon probably in all cases [66] function as mouth-parts (gnathites), and are especially modified for this purpose in Triarthrus, being flattened, shoe-shaped in outline, and so arranged that they work over one another in a shearing fashion. While the more anterior of the coxopodites are attached in front of the posterior tip of the hypostoma, the gnathites of Triarthrus bend backward so that all are behind the hypostoma. In Calymene and Ceraurus, two or three pairs of the gnathites are back of the hypostoma, and one or more pairs may be beside or under the hypostoma. In these genera the mouth is probably in front of the tip of the upper lip. In Isotelus, the mouth seems to have been situated in the notch between the two branches of the hypostoma, and the gnathites of two or three pairs of the appendages probably worked under its forks. Since the length of the hypostoma differs in the various species of Isotelus, there would be a variable number of gnathites projecting under its forks, according to the species. In this genus the gnathites are of the same long form, cylindrical in cross-section, as the endobases of the thoracic segments, but each is bowed back considerably from the point of attachment.
The gnathites of Neolenus are like the endobases of the thorax, but broader. The great length of the hypostoma makes it probable that the mouth was far back and that some of the gnathites were in front of it. The gnathites of Cryptolithus are unknown. Professor Beecher in his drawing shows some fragments with toothed ends near the hypostoma, and it may be that they are inner ends of gnathites, but I see nothing to substantiate such an interpretation. If, as some suppose, Cryptolithus was a mud feeder, the gnathites were probably poorly developed. Of the gnathites of Kootenia, Ptychoparia, and Acidaspis also nothing is known.
In each genus there is a pair of appendages for each segment of the thorax. When the axial lobe is narrow, the endobases of the coxopodites are small and short (Cryptolithus, Ceraurus, Calymene). When the axial lobe is wide, the endobases are long and stout (Isotelus, Triarthrus). The exopodites always lie above and in front of the corresponding endopodites. In Triarthrus the two branches are of practically equal length. In Cryptolithus the exopodites are much the longer. In Neolenus, Calymene, Ceraurus, Kootenia, and Ptychoparia, the exopodites are shorter than the endopodites.
The exopodites in Triarthrus consist of a proximal shaft, succeeded by numerous short segments, and ending distally in a long, grooved, somewhat spatula-shaped segment. Along the anterior margin of the shaft there are many small spines. Along the posterior margin there are numerous flattened setæ, which all lie in one plane and which seem to be more or less united to one another like the barbs of a feather. The setæ are short, not much longer than the width of one of the thoracic segments, and point backward and outward. In Cryptolithus the shaft does not seem to be made up of small segments, and is narrow, with a decided backward curve. The setæ are considerably longer and much more flattened than in Triarthrus. In Calymene the state of preservation does not allow a very full knowledge of the exopodites, but they appear to have a slender, unjointed shaft and short and delicate setæ. The coiled branches of the exopodites as described by Walcott seem to me to be only ordinary Triarthrus-like organs, and this, as I understand from Schuchert, was also the view of Beecher. In Ceraurus the exopodite seems to have been somewhat paddle-shaped, expanded at the distal end, and to have had rather thick, blade-like setæ.
The exopodite of Neolenus is decidedly leaf-like, and reminds one somewhat of the exites [67] of some of the phyllopods. The shaft is a broad unsegmented blade. The setæ are slender, delicate, flattened, and a little longer than the width of the shaft. The exopodites of this genus point forward all along the body. In Kootenia the exopodites are like those of Neolenus, but with a narrower shaft. The exopodites of Ptychoparia appear to be very much like those of Triarthrus, but the shaft is probably not segmented.
The endopodites of the thorax of Triarthrus, Cryptolithus, and Acidaspis show progressive modification from front to back in the broadening of the individual segments and the assumption by them of a triangular form. Not only do the individual segments become more triangular from front to back, but more of the segments of each endopodite become triangular. This modification has so far been seen in these three genera only. The individual segments, except the distal ones, seem to be flattened in all these genera. The distal end of the terminal segment of each endopodite of Triarthrus bears three small movable spines, and each of the segments usually bears three or more spines, located in sockets along the dorsal surface and at the anterior distal angle of each segment. The endopodite of Cryptolithus is bent backward at the carpopodite and this segment is always thickened. At the distal end of the dactylopodite there is a tuft of spines, the triangular segments have tufts of spines on their posterior corners, and there are groups of spines also in the neighborhood of the articulations.
The endopodites of Ceraurus, Calymene, and Isotelus are all relatively slender, the segments are parallel-sided, and there seems to be no particular modification from front to back of the thorax. The endopodites of Isotelus are short, the entire six segments of one being but little longer than the coxopodite of the same appendage. The segments of the endopodites of Neolenus are mostly short and wide, and at the distal end of the terminal segment there are three stout spines. In Kootenia the endopodites are long and very slender. The endopodites of Ptychoparia are too poorly preserved to show details, and those of the thorax of Acidaspis likewise reveal little structure, but they seem to have the triangular modification, and to turn back somewhat sharply at about the position of the carpopodite.
Beecher showed that in Triarthrus there was a pair of appendages on the pygidium for every segment of which it is composed except the last or anal segment (protopygidium). Walcott has since shown that in Neolenus this segment bears a pair of cerci, and Beecher's drawings show that in his later studies he recognized a spinous plate, the possible bearer of cerci, on the anal segment of Triarthrus. The appendages of the anal segment have not yet been seen on other species of trilobites.
The appendages of the pygidium do not show any special modifications, but seem in all cases to be similar to those of the posterior part of the thorax. In Cryptolithus all the pygidial appendages are short and remain beneath the cover of the dorsal test, while in Triarthrus and Neolenus they extend behind it.
In the latter genus the endopodites of the pygidial appendages appear to be practically identical in form with those of the thorax, the individual segments being perhaps a little more nearly square in outline. Like those of the thorax, the segments of the pygidial endopodites bear numerous short spines. The caudal cerci are richly segmented, slightly flexible, spinous tactile organs. They are symmetrically placed, nearly straight when in their natural position, and make an angle of about 75 with one another. They appear to be [68] attached to a narrow rim-like plate which seems to fit in just ahead of the doublure of the pygidium, or perhaps over it.
In Ceraurus, Calymene, and Isotelus, the endopodites of the pygidium are similar to those of the thorax, but seemingly more slender, with less well developed coxopodites, and with, in the last-named genus, slender cylindrical segments. Exopodites are not known on the pygidia of any of these genera, but since they are present and like those of the thorax in Triarthrus, Cryptolithus, Neolenus, and Ptychoparia, there is little reason to think that they were absent in Ceraurus or Calymene, though there is some question about Isotelus.
The limbs are largest and longest on the anterior part of the thorax of a trilobite, and diminish regularly in length and strength to the posterior end of the pygidium. This regular gradation shows, as Beecher was the first to point out, that the growing point of the trilobites is, as in other arthropods, in front of the anal segment. New free segments are introduced into the thorax at the anterior end of the pygidium, and this has led to some confusion between the growing point and the place of introduction of free segments.
If a new segment were introduced at a moult in front of the pygidium, that segment would probably have less fully developed appendages than those adjacent to it, and so make a break in the regular succession. The condition of the appendages corroborates the evidence derived from the ontogeny of the pygidium, and proves that the new segments are introduced at the same growing point as in other Arthropoda.
Bernard, who believed that the Crustacea had been derived through an Apus-like ancestor (1892, pp. 20, 85, 274), pointed out that four or less than four anal cirri were to be expected. Two well developed cirri and two rudimentary ones are present in Apus, and they are also to be found in other phyllopods and some isopods. It is, however, characteristic of the Crustacea as a whole to lack appendages on the anal segment. Caudal cirri (cerci) are much more freely developed in the hexapods than in the Crustacea, particularly in the more primitive orders, Palæodictyoptera, Apterygota, Archiptera, and Neuroptera. They are supposed, in this case, to be modified limbs, and therefore not homologous with the bristles on the anal segment of an annelid. Doctor W. M. Wheeler of the Bussey Institution has kindly allowed me to quote the following excerpt from a letter to me, as expressing the opinion of one who has made an extensive study of the embryology of insects:
I would say that I have no doubt that the cerci of insects are directly inherited from the insect ancestors. They are always highly developed in the lower insects, and only absent or vestigial in a few of the most highly specialized orders such as the Hemiptera, Diptera, and Hymenoptera. I have further no doubt concerning their being originally ambulatory in function. They are certainly not developed independently in insects. Embryologically they arise precisely like the legs, and each cercus contains a diverticulum of the mesoblastic somite precisely as is the case with the ambulatory legs and mouth parts.
The "pygidial antennæ" seem to be as fully developed in Neolenus as in any of the other arthropods, and may suggest a common ancestry of the phyllopods, isopods, and hexapods, in the trilobites. They were doubtless tactile organs, and while the evidence is chiefly negative, it would seem that they proved useless, and were lost early in the phylogeny of this group. Possibly the use of the pygidium as a swimming organ proved destructive to them.
HOMOLOGY OF THE CEPHALIC APPENDAGES WITH THOSE OF OTHER CRUSTACEA.
The head of the typical crustacean bears five pairs of appendages, namely, the antennules, antennas, mandibles, and first and second maxillæ, or, as they are more properly called, the maxillulæ and maxillæ.
As Beecher has pointed out, the "antennæ" of the trilobites, on account of their pre-oral position and invariably uniramous character, are quite certainly to be correlated with the antennules.
The second pair of appendages, the first pair of biramous ones, Beecher homologized with the antennæ of other crustaceans, and that homology has been generally accepted, though Kingsley (1897) suggested that it was possible that no representatives of the true antennæ were present.
In preparing the restorations in the present study, the greatest difficulty has been to adjust the organs about the mouth. In Triarthrus, numerous specimens show that without question there are four pairs of gnathites back of the hypostoma, and that all four belong to the cephalon. In forms with a long hypostoma, however, there was no room on the cephalon for the attachment of four pairs of gnathites, neither were there enough appendifers to supply the requisite fulcra. At first I supposed I had solved the difficulty by assuming the mouth to be in front of the posterior tip of the hypostoma, as it really is in Ceraurus and Calymene, and allowing the gnathites to play under the hypostoma as Walcott (1912) has shown that they do in Marrella. Finally, when I came to study in greater detail the slices of Calymene and Ceraurus, they seemed to show that the anterior one or two pairs of appendages became degenerate and under-developed. This was probably a specialization due to the great development of the hypostoma in trilobites, that organ being much more prominent in this than in any other group. As the hypostoma lengthened to accommodate the increasing size of sub-glabellar organs (stomach, heart, etc.), the mouth migrated backward, leaving the anterior appendages ahead of it, with their gnathobases, at least, functionless. That such migration has taken place, even in Triarthrus, is shown by the fact that the points of articulation of the first biramous appendages are pre-oral, and it is more obviously true of Ceraurus. Correlated with the weakening of the appendages on the lower surface is the loss of glabellar furrows on the upper surface. The glabellar furrows mark lines of infolding of the test to form the appendifers and other rugosities for the attachment of tendons and muscles. It is conceivable that this migration backward of the mouth began very early in the history of the race, and that even before Cambrian times, the antennæ, probably originally biramous appendages like those on the remainder of the body, had dwindled away and become lost. If this is the case, then the first pair of biramous appendages of Triarthrus would be mandibles, the second pair maxillulæ, and the third pair maxillæ.
There remain the last pair of cephalic appendages, and they bring up the whole head problem of the trilobites. Beecher has stated (1897 A, p. 96) his conviction that the head of the trilobite is made up of five segments, representing the third, fourth, fifth, sixth, and seventh neuromeres of the theoretical crustacean. As a matter of fact, he really made up the head of seven segments, since he stated that the first neuromere was represented by the hypostoma and the second by the epistoma and free cheeks.
Jaekel (1901, p. 157) nearly agreed with Beecher, but made eight segments, as he saw [70] five segments in the glabella of certain trilobites. In his table (p. 165) he has listed the segments with their appendages as follows: 1. Acron, with hypostoma; 2, rostrum (epistoma), with free cheeks; 3, first frontal lobe, with (?) antennules; 4, second frontal lobe, with antennæ; 5, mandibles; 6, first, or pre-maxillæ; 7, second maxillæ; 8, occipital segment with maxillipeds.
Jaekel refused to believe that the antennæ of trilobites were really entirely simple, and so homologized them with the antennæ and not the antennules of other Crustacea. In this he was obviously incorrect, but it accounts for his homology of the remainder of the cephalic appendages.
It is, at present, impossible to demonstrate the actual number of somites in the cephalon of the trilobite, but I believe that Beecher was correct in holding that the glabella was composed of four segments. There are, it is true, a number of trilobites (Mesonacidæ, Paradoxidæ Cheiruridæ, etc.) which show distinctly four pairs of glabellar furrows, indicating five segments in the glabella. This is, however, probably due to a secondary division of the first lobe.
The correspondence of the five segments on the dorsal side with the five pairs of appendages makes it unlikely that any pair of limbs has been lost. The condition in Marrella, where a trilobite-like cephalon bears five pairs of appendages, the second pair of which are tactile antennæ, is favorable to the above interpretation. In spite of the apparent degeneration of the first two pairs of appendages in Calymene, no limbs are actually missing, and if some are dropped out in the later trilobites it would not affect the homology of those now known. I therefore agree with Beecher in homologizing the appendages, pair for pair, with those of the higher Crustacea.
The antennules were obviously tactile organs, probably freely movable in most trilobites, but in the case of Triarthrus perhaps rather rigid, judging from the great numbers of specimens which show the characteristic sigmoid curve made familiar by Professor Beecher's restoration. The proximal end of the shaft of each antennule of Triarthrus is hemispheric and doubtless fitted into a socket, thus suggesting great mobility of the whole organ. In spite of this, I have seen no specimens in which they did not turn in toward each other and cross the anterior margin very near the median line. In front of the margin, various specimens show evidence of flexibility, but from the proximal end to the margin the position is the same in all specimens.
In all the few specimens of Cryptolithus retaining the antennules, these organs are turned directly backward, but it is entirely within the range of probabilities that while its burrowing habits made this the more usual position, the animal had the power of turning them around to the front when they could be used to advantage in that direction.
It has been the opinion of most observers that the exopodites of trilobites were swimming organs, while others have thought that they functioned also in aerating the blood. To the present writer it seems probable that the chief function was that of acting as gills, for which the numerous thin, flattened or blade-like setæ are particularly adapted. That [71] they were also used in swimming is of course possible, but that was not their chief function. It should be remembered that the exopodites are always found dorsal to or above the endopodites, and in a horizontal plane. For use in swimming it would have been necessary to rotate each exopodite into a plane approximately perpendicular to or at least making a considerable angle with the dorsal test. In this position, the exopodites would have been thrust down between the endopodites, and one would expect to find some specimens in which a part at least of the exopodites were ventral to the endopodites. Specimens in this condition have not yet been seen among the fossils. To avoid having the exopodites and endopodites intermingled in this way, the animal would have to bring all the endopodites together along the axial line in a plane approximately perpendicular to the dorsal test, in which case the exopodites would be free to act as swimming organs. The fact that the setæ of an exopodite stay together like the barbs on a feather would of course tend to strengthen the idea that the exopodites could be used in swimming, but that is not the only possible explanation of this condition. The union of the basipodite and exopodite shows that the two branches of the appendage acted together. Every movement of one affected the other, and the motion of the endopodites in either swimming or crawling produced a movement of the exopodites which helped to keep up a circulation of water, thus insuring a constant supply of oxygen.
Although Neolenus is usually accounted a less primitive form than Ptychoparia or Triarthrus, it has much the most primitive type of exopodite yet known. It would appear that the exopodites were originally broad, thin, simple lamellæ, which became broken up, on the posterior side, into fine cylindrical setæ. As development progressed, more and more of the original lamella was broken up until there remained only the anterior margin, which became thickened and strengthened to support the delicate filaments. The setæ in turn became modified from their original simple cylindrical shape to form the wide, thin, blade-like filaments of Cryptolithus and Ceraurus.
Another possible use of the exopodites is suggested by the action of some of the barnacles, which use similar organs as nets in gathering food and the endopodites as rakes which take off the particles and convey them to the mouth. The exopodites of the trilobite might well set up currents which would direct food into the median groove, where it could be carried forward to the mouth.
The endopodites were undoubtedly used for crawling; in some trilobites, probably most of them, for swimming; in the case of Cryptolithus, and probably others, for burrowing; and probably in all for gathering food, in which function the numerous spines with which they are arrayed doubtless assisted.
Various trails have been ascribed to the action of trilobites, and many of them doubtless were made by those animals (see especially Walcott, 1918). Some of these trails seem to indicate that in crawling the animal rested on the greater part of each endopodite, while others, notably the Protichnites recently interpreted by Walcott (1912 B, p. 275, pl. 47), seem to have touched only the spinous tips of the dactylopodites to the substratum. The question of the tracks, trails, and burrows which have been ascribed to trilobites is discussed briefly on a later page; but can not be taken up fully, as it would require another monograph to treat of them satisfactorily.
The flattened, more or less triangular segments of the endopodites of the posterior part [72] of the thorax and pygidium in Triarthrus, Cryptolithus, and Acidaspis probably show an adaptation of the endopodites of the posterior part of the body both as more efficient pushing organs and as better swimming legs. The fact that these segments are pointed below enabled them to get a better grip on whatever they were crawling over, and the flattening allowed a much greater surface to be opposed to the water in swimming. In this connection it might be stated that it seems very probable that the trilobites with large pygidia at least, perhaps all trilobites, had longitudinal muscles which allowed them to swim by an up and down motion of the fin-like posterior shield, the pygidium acting like the caudal fin of a squid. Such a use would explain the function of the large, nearly flat pygidia seen in so many of the trilobites beginning with the Middle Cambrian, and of those with wide concave borders. It should be noted here that it is in trilobites like Isotelus, with pygidia particularly adapted to this method of swimming, that the endopodites are most feebly developed, and show no flattening or modification as swimming organs.
The relatively strong, curved, bristle-studded endopodites of Cryptolithus, combined with its shovel-shaped cephalon, indicate Limulus-like burrowing habits for the animal, and the mud-filled casts of its intestine corroborate this view. That it was not, however, entirely a mud groveller is indicated by its widespread distribution in middle Ordovician times.
Use of the Pygidium in Swimming.
The idea that the use of the pygidium as a swimming organ is a possible explanation of that caudalization which is so characteristic of trilobites has not been developed so far as its merits seem to deserve. Two principal uses for a large pygidium of course occur to one: either it might form a sort of operculum to complete the protection when the trilobite was enrolled, or it might serve as a swimming organ. That the former was one of its important functions is shown by the nicety with which the cephalon and pygidium are adapted to one another in such families as the Agnostidæ, Asaphidæ, Phacopidæ, and others. That a large pygidium is not essential to perfect protection on enrollment is shown by an equally perfect adjustment of the two shields in some families with small pygidia, notably the Harpedidæ and Cheiruridæ That the large pygidial shields are not for protective purposes only is also shown by those forms with large pygidia which are not adjusted to the conformation of the cephalon, as in the Goldiidæ and Lichadidæ. It is evident that a large pygidium, while useful to complete protection on enrollment, is not essential.
It would probably be impossible to demonstrate that the trilobites used the pygidium in swimming. The following facts may, however, be brought forward as indicating that they probably did so use them.
1. The appendages, both exopodites and endopodites, are relatively feebly developed as swimming organs. This has been discussed above, and need not be repeated. It must in fairness be observed, however, that many modern Crustacea get about very well with limbs no better adapted for swimming than those of the trilobites.
2. The articulations of the thoracic segments with each other and with the two shields are such as to allow the pygidium to swing through an arc of at least 270, that is, from a position above the body and at right angles to it, around to the plane of the bottom of the cephalon. Specimens are occasionally found in which the thorax and pygidium are so flexed that the latter shield stands straight above the body. A well preserved Dipleura in this position is on exhibition in the Museum of Comparative Zoology, and Mr. Narraway and I have figured a Bumastus milleri in the same attitude (Ann. Carnegie Mus., vol. 4, 1908, pl. 62, fig. 3).
3. What little can be learned of the musculature (see under musculature, seq.) indicates that the trilobites had powerful extensor and flexor muscles, such as would be required for this method of swimming. It may be objected that the longitudinal muscles were too small to permit the use of a caudal fin. In the lobster, where this method of progression is most highly developed, there is a large mass of muscular tissue which nearly fills the posterior segments. Trilobites have not usually been thought of as powerfully muscled, but it may be noted that in many cases broad axial lobes accompany large pygidia. As the chief digestive region appears to have been at the anterior end, and other organs are not largely developed, it seems probable that the great enlargement of the axial lobe was to accommodate the increased muscles necessary to properly operate the pygidium. It may be noted that in all these genera the axial lobe of the pygidium is either short or narrow.
4. The geological history of the rise of caudalization favors this view. With the exception of the Agnostidæ and Eodiscidæ, all Lower Cambrian trilobites had small pygidia, and the same is true of those of the Middle Cambrian of the Atlantic realm (except for the Dorypyge of Bornholm). In Pacific seas, however, large-tailed trilobites of the families Oryctocephalidæ, Bathyuridæ, and Asaphidæ then began to be fairly common, though making up but a small part of the total fauna of trilobites. In the Upper Cambrian of the Atlantic province the Agnostidæ were the sole representatives of the isopygous trilobites, while in the Pacific still another family, the Dikelocephalidæ, was added to those previously existing.
With the Ordovician, caudalization reached its climax and the fashion swept all over the world. It is shown not so much in the proportion of families with large pygidia, as in the very great development of the particular trilobites so equipped. Asaphidæ and Illænidæ were then dominant, and the Proëtidæ, Cyclopygidæ Goldiidæ, and Lichadidæ had begun their existence. A similar story is told by the Silurian record, except that the burden of the Asaphidæ has been transferred to the Lichadidæ and Goldiidæ. All the really old (Cambrian) families of trilobites with small pygidia had now disappeared. In the general dwindling of the subclass through the Devonian and later Palæozoic, the few surviving species with small pygidia were the first to go, and the proëtids with large abdominal shields the last.
The explanation of this history is probably to be found in the rise of the predatory cephalopods and fishes, the natural enemies of the trilobites, against whom they could have no other protection than their agility in escaping. While the records at present known carry the fishes back only so far as the Ordovician (fishes may have arisen in fresh waters and have gone to sea in a limited way in the Ordovician and more so in Silurian time) and the cephalopods to the Upper Cambrian, the rise of the latter must have begun at an earlier date, and it is probably no more than fair to conjecture that the attempt to escape swimming enemies caused an increase in the swimming powers of the trilobites themselves. At any rate, the time of the great development of the straight cephalopods coincided with the time of greatest development of caudalization; both were initiated in the Pacific realm, and both spread throughout the marine world during the middle Ordovician. And since, in the asaphids, a decrease in swimming power of the appendages accompanied the increase in the size of the pygidium, it seems probable that the swimming function of the one had been transferred to the other. A high-speed, erratic motion which could be produced by the sudden flap of a pygidium would be of more service in escape than any amount of steady swiftness produced by the oar-like appendages of an animal of the shape of a trilobite.
The primary function of the endobases of the coxopodites was doubtless the gathering, preparation, and carrying of food to the mouth. Although the endobases of opposite sides could not in all cases meet one another, they were probably spinose or setiferous and could readily pass food from any part of the axial groove forward to the mouth, and also send it in currents of water. The endobases of the cephalic coxopodites were probably modified as gnathites in all cases, but little is known of them except in Triarthrus, where they were flattened and worked over one another so as to make excellent shears for slicing up food, either animal or vegetable. In some cases the proximal ends of opposed gnathites were toothed so as to act as jaws, but a great deal still remains to be learned about the oral organs of all species.
The writer has suggested (1910, p. 131) that a secondary function of the endobases of the thorax of Isotelus and probably other trilobites with wide axial lobes was that of locomotion. In Isotelus the endobases of the thorax are greatly over-developed, each being much stouter and nearly as long as the corresponding endopodite, and the explanation seemed to me to lie in the locomotor or crawling use of these organs instead of the endopodites. Certain trails which I figured seemed to support this view.
POSITION OF THE APPENDAGES IN LIFE.
In almost all the specimens so far recovered the appendages are either flattened by pressure or lie with their flat surfaces in or very near the plane of stratification of the sediment. This flattening is extreme in Neolenus, Ptychoparia, and Kootenia, moderate in Triarthrus and Cryptolithus, and apparently slight or not effective in Isotelus, Ceraurus, and Calymene. These last are, however, from the conditions of preservation, least available for study.
In Part IV, attention is called to a specimen of Triarthrus (No. 222) in which some of the endopodites are imbedded nearly at right angles to the stratification of the shale. This specimen is especially valuable because it shows that the appendages in the average specimen of Triarthrus have suffered very little compression, and it also suggests the probable position of the endopodites when used for crawling.
In considering the position of the appendages in life, one must always remember one great outstanding feature of trilobites, the thinness and flexibility of the ventral membrane. The appendages were not inserted in any rigid test but were held only by muscular and connective tissue. Hence we must premise for them great freedom of motion, and also relatively little power. The rigid appendifers, and the supporting apodemes discovered by Beecher, supplied fulcra against which they could push, but their attachment to these was rather loose.
Considering, first, the position of the appendages in crawling, it appears that different trilobites used their appendages in different ways. Neolenus had compact stocky legs, which allowed little play of one segment on another, as is shown by the wide joints at right angles to the axis of the segment. Such limbs were stiff enough to support the body when the animal was crawling beneath the water, where of course it weighed but little. That such a crawling attitude was adopted by trilobites has been shown by Walcott in his explanation of the trails known as Protichnites (1912 B, p. 278). Many trilobites probably crawled in [75] this way, on the tips of the toes, so to speak. In such the limbs would probably extend downward and outward, with the flattened sides vertical.
The limb of Triarthrus, however, is of another type. The endopodites are long, slender, flexibly jointed, the whole endopodite probably too flexible to be used as a unit as a leg must be in walking on the "toes." The proximal segments of the thoracic and pygidial endopodites are, however, triangular instead of straight-sided, and, the spine-bearing apex of the triangle being ventral, it enabled the endopodites to get a grip on the bottom and thus push the animal forward. This method of progression was more clumsy and less rapid than that of Neolenus, but it sufficed. The natural position of the endopodite when used in this way would seem to be with the flattened sides of the segments standing at an angle of 30 to 45 with the vertical, thus allowing a good purchase on the bottom and at the same time offering the minimum resistance to the water when moving the appendages forward.
Isotelus has endopodites different from those of either Neolenus or Triarthrus. They are composed of cylindrical segments, the joints indicating a certain amount of flexibility. Since there is no method by which the segments may get a purchase on the bottom other than by pushing with the distal ends, it would seem at first thought that Isotelus, like Neolenus, crawled on its "toes." The endopodites of Isotelus are however, short and feeble when compared with the size of the test, while the endobases of the coxopodites are extraordinarily developed. These facts, together with certain trails, strongly suggest the use of the coxopodites as the primary ambulatory organs, the endopodites probably assisting. In this event, the position of the endopodites and coxopodites would be downward, both outward and inward from the point of attachment, and the motion both backward and forward. The fact that in the specimens as preserved the coxopodites point backward and the endopodites forward indicates that the limb as a whole swung on a pivot at the appendifer. It is of course natural to suggest that the coxopodites and endopodites of all the trilobites with wide axial lobes, Nileus, Bumastus, Homalonotus, etc., were developed in this same way.
Cryptolithus presents still another and very peculiar development of the endopodites where ability to get purchase on the sea floor is obtained by a stout limb of slight flexibility, bowed and turned backward in the middle, where an enlarged segment insures stiffness. The segments are flattened, and since the greatest strength when used in pushing and crawling is in the long axis of the oval section of the flattened limb, it seems probable that these limbs did not hang directly down, with their sides vertical, but that their position in life was very much the same as that in which they are preserved as fossils. By moving these bowed legs forward and backward in a plane at a small angle to the surface of the body, a powerful pushing impetus could be obtained. They may, however, have occupied much the same position as do those of Limulus.
In the case of the endopodites, therefore, it is necessary to study the structure and probable method of their use in each individual genus before suggesting what was the probable position in life. In the act of swimming, the position was probably more uniform. When the endopodites were used in swimming, as they undoubtedly could be with more or less effect in all the trilobites now known, those with flattened surfaces probably had them at such an angle as to give the best push against the water on the back stroke, while on the forward stroke the appendage would be turned so that' the thin edge opposed the water. The great flexibility of attachment would certainly permit this, though unfortunately nothing [76] is as yet known of the musculature. The coxopodites of course had less freedom of movement in this respect, and probably could not turn their faces. For this reason, it seems to me likely that those coxopodites which are compressed did not stand with their flattened faces vertical, but in a position which was nearly horizontal or at least not more than 45 from the horizontal. If the flattened faces were vertical, they would be in constant opposition to the water during forward movements and would be of no use in setting up currents of water toward the mouth, as every back stroke would reverse the motion.
The position of the exopodites in life seems to have been rather uniform in all the genera now known. I have set forth on a previous page my reasons for thinking that they took little part in swimming, and I look upon them as being, in effect, leaf-gills. It seems probable that in all genera the exopodites were held rather close to the test, the shaft more or less rigid, the filamentous setæ gracefully pendent, but pendent as a sheet and not individually, there having been some method by which adjoining setæ were connected laterally. Free contact with the water was thus obtained without the mingling of endopodites and exopodites which would have been so disastrous to progression.
PART II.
Structure And Habits Of Trilobites.
Granting that the trilobite is a simple, generalized, ancient crustacean, it appears justifiable to attribute to it such internal organs as seem, from a study of comparative anatomy, to be primitive.
The alimentary canal would be expected to be straight and simple, curving downward to the mouth, and should be composed of three portions, stomodæum, mesenteron, and proctodæum, the first and last with chitinous lining. In modern Crustacea, muscle-bands run from the gut to part of the adjacent body wall, so that scars of attachment of these muscles may be sought. At the anterior end of the stomodæum, they are usually especially strong. From the mesenteron there might be pouch-like or tubular outgrowths.
The heart would probably be long and tubular, with a pair of ostia for each somite.
In modern Crustacea, the chief organs of renal excretion are two pairs of glands in the head, one lying at the base of the antennæ and one at the base of the maxillæ. Only one pair is functional at a time, but these are supposed to be survivors of a series of segmentally arranged organs, so that there might be a pair to each somite of a trilobite.
The nervous system might be expected to consist of a supracesophageal "brain," comprising at least two pairs of ganglionic centers, and a double ventral chain of ganglia with a ladder-like arrangement.
Besides these organs, a variety of glands of special function might be predicted.
Reproductive organs probably should occur in pairs, and more than one pair is to be expected. There is little to indicate the probable location of the genital openings, but they may have been located all along the body back of the cephalon.
It may be profitable to summarize present knowledge of such traces of these organs as have been found in the fossils, if only to point out what should be sought.
Beyrich (1846, p. 30) first called attention to the alimentary canal of a trilobite, (Cryptolithus goldfussi,) and Barrande (1852, p. 229) confirmed his observations. A number of specimens of this species have been found which show a straight cylindrical tube or its filling, extending from the glabella back nearly to the posterior end of the pygidium. It lies directly under the median line of the axial lobe, and less than its own diameter beneath the dorsal test. At the anterior end it apparently enlarges to occupy the greater part of the space between the glabella and the hypostoma, but was said by the early observers to extend only a little over halfway to the front. Beyrich thought the position of the median tubercle indicated the location of the anterior end.
Walcott (1881, p. 200) stated that in his experience in cutting sections of trilobites it was a very rare occurrence to find traces of the alimentary canal. The visceral cavity was usually filled with crystalline calcite and all vestiges of organs obliterated. There were, however, some slices which showed a dark spot under the axial lobe, which probably represented the canal. In his restoration he showed it as of practically uniform diameter throughout, and extending but slightly in front of the mouth.
Jaekel (1901, p. 168, fig. 28) has produced a very different restoration. His discussion of this point seems so good, and has been so completely overlooked, that I will append a slightly abridged version of a translation made some years ago for Professor Beecher. The idea was, however, not original with Jaekel, as it was suggested by Bernard (1894, p. 417), but not worked out in detail.
While considering the problem as to what organ could have lain beneath the glabella of the trilobite, and while studying the organization of living Crustacea for the purpose of comparison, I found in the collections of the Geological Institute preparations of Limulus which seemed to me to directly solve the entire question.
From the mouth, which lies at about the middle of the head shield, the œsophagus bends forward, swells out at the frontal margin of the animal at a sharp upward bend in order to take a straight course backward after the formation of an enlarged stomach. Still within the head shield there branch out from each' side of the canal two small vessels which pass over into the richly branched mass of liver lying under the broad lateral parts of the head shield. After seeing this specimen, I no longer had the least doubt that the head shield of the trilobites is to be interpreted in a similar manner. The position of the hypostoma and gnathopods makes it necessary to assume that the position of the mouth of the trilobite lay pretty far back. If, therefore, this depends upon the secondary ventral deflection of the oral region, as seems to be the case, then it is a priori probable that the anterior part of the canal has also shared in this ventral inflection.
The posterior part of the canal in the region of the segmented thorax and pygidium is comparatively narrow, as shown long ago by Beyrich; he represents only a thin tube which shows no swellings whatever, and such are usually missing in Arthropoda.
As the glabella of most trilobites is regularly convex, there must lie beneath it an organ running from front to back, which presses the bases of the cephalic legs away from each other and down from the dorsal test. An organ so extensive and unpaired, running thus from front to back, can, among the Arthropoda, be regarded only as an alimentary canal, for the swellings of the cephalic ganglia and the heart are by far too small to produce such striking elevations on the front and upper surface of the glabella. The canal might then have consisted of a gizzard belonging to the oesophagus, and a stomach proper or main digestive canal.
… Among the trilobites there are two pairs of vessels on both sides of the glabella which have precisely the same position with reference to the supposed course of the alimentary canal as the ducts of the hepatic lobes in Limulus. One observes in numerous trilobites, although in different degrees of clearness and under various modifications, a dendritic marking of the inner surface of the cheeks which takes its rise at the lateral margins of the glabella and spreads thence like a bush over the entire surface of the cheeks. Exactly the same position is taken by the richly branched hepatic lobes of Limulus on the lower surface of the head shield; a fact of special weight in favor of the homology and similar significance of the two phenomena, is that in the trilobites also, the anterior of the two main ducts is the larger, the posterior the smaller. The striking similarity of the two structures is shown by a comparison of the head shield of Eurycare [Elyx] from the Cambrian of Sweden, in which the course of the canals is shown with remarkable clearness [with those of Limulus].
I have been able to convince myself that the existence of the two canals on each side is also the rule in other genera, even though the posterior pair is frequently but feebly developed or completely obscured by the anterior pair. In Dionide formosa, for example, I find only the anterior pair, which is very large and divided into two principal branches. From all these considerations it seems to me no longer doubtful that the median elevation was caused by the stomach and gizzard, and that the cheeks have principally served to cover the hepatic appendages of the alimentary canal.
The cause of the incomplete development of the glabellar lobes lies, hence, in the intrusion of the alimentary canal, and it makes naturally the most effect where the gizzard spreads out and bends into the stomach. This spot lies behind the frontal lobe, which is hence increased in size according as the stomach increases in size; in this way not only the foremost segments of the glabella become enlarged, but also the following ones more or less pressed aside. This process is easily followed phylogenetically and ontogenetically.
From the latter point of view, the development of Paradoxides is very instructive. In a head shield 2.5 mm. long the whole anterior part of the glabella is broadened, but the five pairs of lateral impressions are clearly marked and the six segments of the head bounded by them are all of about the same size. In a head shield about 13 mm. long, the foremost segment is very much increased in size, the jaw lobes pressed still further apart; in adult forms both anterior segments are combined into the frontal swellings of the glabella. In other groups this process proceeds phylogenetically still further, so that among the Phacopidæ and in Trinucleus, behind the frontal swelling of the glabella only the last cephalic segment retains a certain independence. The frontal lobe is thus no definite part, although it is as a rule composed of the mesotergites of the first two cranidial segments.
This idea of an enlarged mesenteron certainly has much to commend it, and such actual evidence as exists seems in favor of rather than against it. The strongest, firmest, best-protected place in the whole body of the trilobite is the cavity between the vaulted glabella and the hypostoma. As Jaekel has said, it is far too large a cavity for the brain, larger than would seem to be required for a heart, and what else could be there but a stomach? As has already been pointed out, Beyrich and Barrande found a pear-shaped enlargement of the alimentary canal under the glabella of Cryptolithus. Longitudinal sections through the glabella of Calymene and Ceraurus practically always show the cavity there filled with clear crystalline calcite. One actual specimen of Ceraurus (Walcott 1881, pl. 4, fig. 1) shows the cavity between the glabella and hypostoma entirely empty. The vacant spaces in these two classes of specimens do not, however, necessarily mean anything more than imperfect preservation.
This species is taken up first, as it is the one shown in Walcott's often-copied figure (1881, pl. 4, fig. 6). It is to be feared that too many have looked at this figure without reading the accompanying explanation, and have taken it for a copy of an actual specimen and not a mere diagram, which it admittedly is. The evidence on which it is based is comprised in eight transverse slices, one through the glabella and seven through the thorax. Three of these have been figured by Walcott: No. 27, 1881, pl. 3, fig. 7; No. 13, 1881, pl. 2, fig. 3, 1918, pl. 26, fig. 14; No. 202, 1918, pl. 27, fig. 8. In all, as can be seen by reference to the figures, the canal is partially collapsed, and is much larger than is indicated in Walcott's restoration. The other sections bear out the testimony of those figured. One of these figured specimens (No. 27) and another figured herewith (No. 118, see fig. 21) show an exceedingly interesting structure which has previously escaped notice. The body cavity seems to have had, in this region at least, a chitinous sheath on the dorsal side. As shown especially in figure 21, this sheath impinges dorsally and laterally against the axial lobe and thus furnishes a special protection for the soft organs beneath, probably protecting them from the strain of the dorsal muscles.
While there is no way in which the location of these sections in the thorax can be positively determined, it is probable that they came from the anterior end. In sections further back, supposed to be in the posterior region of the mesenteron, no sheath is shown, but the canal is nearly if not quite as large in relation to the size of the axial lobe.
The single section through the glabella (specimen 97) is of course important and fortunately well preserved (fig. 22). It shows the dorsal sheath pressed against the inner surface of the axial lobe along its middle portion, but diverging from it at the sides. The section of the canal is oval, nearly twice as wide as high, but it is obviously somewhat depressed. The original canal evidently filled nearly the whole of the dorsal part of the glabella in this particular region. Unfortunately, the connection with the mouth is not shown, and the form of the hypostoma indicates that the section cut the glabella diagonally, either in the anterior or posterior part, probably the latter. In all these cases it should be remembered that the specimens were found lying on their backs, and the canal has fallen in (dorsally) since death.
The sections show that in Ceraurus pleurexanthemus the anterior part of the alimentary canal was large, filling the part of the glabella below the heart; that the body cavity was provided with a chitinous dorsal sheath extending back into the thorax; and that the posterior portion of the mesenteron was likewise large and oval in section. Since the alimentary canal must be connected with the mouth and anus, some such restoration as that of Jaekel is indicated. No chitinous lining of the stomodæum or proctodæum was found, but it is not certain that any of the sections cut either of those regions.
Ten transverse sections and one longitudinal slice show the form of the alimentary canal in Calymene. One of these has been figured by Walcott (1881, pl. 1, fig. 9) but without showing the organ in question.
The only section cutting the cephalon which shows any trace of the canal is a longitudinal one (No. 141), which is not very satisfactory. It has a large, nearly circular, opaque spot under the anterior part of the glabella which may or may not represent a section across the anterior end of the mesenteron. Three sections (No. 9, 115, 143) show the dorsal sheath, the latter having the mud-filled canal beneath it. The sheath arches across the axial lobe as in Ceraurus, leaving room for the dorsal muscles at the sides and above it. In this region the canal is large and oval in section. Six slices cut the mesenteron behind the abdominal sheath (Nos. 39, 117, 148, 153, 62, 65) (see fig. 23). In the first four of these it is oval in section and large, but not so large as in No. 143. In the last two, it is small and circular in section, from which it is inferred that the canal tapers posteriorly.
Cryptolithus goldfussi (Barrande).
Illustrated: Beyrich, Untersuch. über Trilobiten, Berlin, 1846, pl. 4, fig. 1c.—Barrande, Syst. Sil. Bohême, vol. 1 1852, pl. 30, figs. 38, 39.
Both Beyrich and Barrande have shown that from the posterior end of the axial lobe to the neck-ring on the cephalon, the alimentary canal in Cryptolithus has a nearly uniform diameter of less than half the width of the axial lobe. In front of the neck-ring, it enlarges, and while its original describers state that it extends only about halfway to the front of [81] the glabella, Barrande's figure 39 shows it extending quite to the front, and his figure 38 shows it fully two thirds of the distance to the anterior end, as does Beyrich's figure of 1846.
The Museum of Comparative Zoology contains a single specimen of this species from Wesela, Bohemia, which shows the course of the canal from the middle of the pygidium to the anterior part of the glabella. The enlargement appears to begin about halfway to the front of the glabella and to be greatest at the anterior end. At the anterior end of the glabella, the anterior end of the thorax, and the posterior end of the pygidium, the canal is still packed full of a material somewhat darker in appearance than the matrix, while the remainder of it is open. A well defined constriction is present under the middle of the next to the last thoracic segment, but whether this is accidental or whether it indicates the point where the mesenteron discharges into the proctodæum can not be determined. The inside of the canal has somewhat of a lustre and there are three conical projections into it on the median ventral line, a very small one in front of the neck furrow, a larger one under the anterior part of the second segment, and a third between the fourth and fifth segments.
The specimens of Cryptolithus from Bohemia and of Ceraurus and Calymene from New York seem to substantiate the claim of Bernard and Jaekel that at the anterior end of the canal there was an enlarged organ which occupied the greater part of the cavity of the glabella. It appears that it extended into the thorax, and that above it and the heart was a chitinous dorsal sheath. Behind the enlarged portion, the mesenteron appears to have been of practically uniform diameter in Cryptolithus, but to have tapered posteriorly in Ceraurus and Calymene. The proctodæum can not yet be differentiated from the mesenteron, and only in Cryptolithus has the posterior portion of the alimentary canal been seen. It is, there, merely a continuation of the mesenteron. The stomodæum likewise has not been identified, but was probably a short gullet leading up from the mouth into the enlarged digestive cavity.
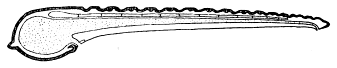
Fig. 24. Longitudinal section of Ceraurus pleurexanthemus, showing the probable outline of the alimentary canal and the heart above it. A restoration based on the slices described above.
The principle of the enlargement of the latter and its influence on the dorsal shell once established, the significance of different types of glabellæ becomes apparent. It will be remembered that the glabella of the protaspis of most trilobites is narrow, and that the same is true of the glabellæ of most ancient and all primitive trilobites. The free-swimming larvæ and the free-swimming ancestors of the trilobites were probably strictly carnivorous, lived on concentrated food, and needed but a small digestive tract. As the animals "discovered the ocean bottom" and began to be omnivorous or herbivorous, larger stomachs were required, and so in the later and more specialized trilobites the glabella became expanded latterally or dorsally, or both, to meet the requirement for more space, until, in such Devonian genera as Phacops, the cephalon was nearly all glabella.
Jaekel's suggestion, quoted above, that the so called "nervures" seen on the under surfaces of the heads of some trilobites are really glands for the secretion of digestive juices, is at least worthy of consideration. Moberg, however (1902, p. 299), suggested that these markings probably had something to do with the eyes rather than the stomach. He says in part (translation):
In general we can now say that such features are common to all the eyeless Conocoryphidæ. With the conocoryphs I include Elyx and consider Harpides as at least closely related. Similar impressions are also found in forms with eyes, as, for instance, in the Olenidæ, but here such radiate partly from the border of the eye, partly from the front end of the glabella, partly from the [visual surface of the] eye, and sometimes from the angle between the occipital ring and the glabella. They therefore go out from such different points that they can not possibly be branches of the liver. It would also be very remarkable if such an important organ should have been developed in a few eyeless forms, but have failed to leave the least trace in the rest of the trilobites.
Lindstroem (1901, pp. 18, 19, 33; pl. 5. figs. 29, 31; pl. 6, figs. 43-45) has discussed these markings and given beautiful figures showing their appearance in Olenus, Parabolina, Elyx, Conocoryphe, and Solenopleura. He decided that they were to be explained as branches of the circulatory system, comparing them with the veins and arteries of Limulus. He pointed out that there was a coincidence between these markings and the position of the eyes, and suggested a causal connection with the latter.
Beecher (1895 B, p. 309), also from a comparison with Limulus, suggested that the eye-lines of Cryptolithus, Harpes, Conocoryphe, Olenus, Ptychoparia, Arethusina, etc., probably represented the optic nerves, and since the eye-lines are usually the main trunks of the dendritic markings, it is fair to assume that he considered the whole as due to branches of nerves.
Reed has recently (1916, pp. 122, 173) discussed these lines as developed in the Trinucleidæ, and seems to accept Beecher's explanation.
Three explanations of the "nervures" are thus current, and the authors of all of them refer us to Limulus as proving their claims! So far as general appearance goes, the markings on the trilobites more closely resemble the veins of a Limulus than either the nerves or "liver" of that animal. The veins, however, are not in contact with the dorsal shell, but are buried in the liver and muscles, while the arrangement of the arteries, which are dorsal in position, is quite unlike what is seen in the trilobites.
The term nervures, as applied to these markings, is not only misleading, but an incorrect use of one of Barrande's words, for by nervures he meant delicate surface markings. Until the real function of the organs which made these markings is definitely established, it may be well to call them genal cæca, for they obviously were open tunnels ending blindly, whatever they contained.
The question of the function of the genal cæca can not, in any case, be settled by an appeal to Limulus, and it is doubtful if it can be settled at all at the present time. Certain things tend to show that Jacket's explanation is the most plausible, and these may be briefly set forth.
Walcott (1912 A, pp. 176, 179, pls. 27, 28) has described specimens of Naraoia and Burgessia in which similar markings are well shown, and where they are obviously connected with the alimentary canal just at the anterior end of the mesenteron. In Burgessia, which seems to be a notostracan branchiopod, the trunk sinuses are very wide, and the appearance [83] is on the whole unlike that of any known trilobite. In Naraoia, however, the markings are much finer and directly comparable with those of Elyx. If my contention that Naraoia is a trilobite should be sustained, it might almost settle the question of the "nervures." In Burgessia these lateral trunks enter the main canal behind the fifth pair of appendages. In the trilobites they debouch much further forward.
The principal argument in favor of the interpretation of these markings as nerves lies in their connection with the eyes. There is considerable evidence to indicate that the eye-lines and the genal cæca are two distinct structures, but because both originate from the sides of the anterior lobe of the glabella, and both extend outward at nearly right angles to the axis, or obliquely backward, they are, when both present, coincident. Genal cæca occur on blind trilobites, on trilobites with simple eyes, and on trilobites with compound eyes. Eye-lines occur on trilobites with both simple and compound eyes, and genal cæca may or may not be present in both cases. The morphology of the ridge forming the eye-line in trilobites with compound eyes is well known. It is abundantly proved by ontogeny that it is the continuation of the palpebral lobe, and a development of the pleura of the first dorsal segment of the cephalon. Lake, Swinnerton, and Reed have tried to show that the eye-lines of the Harpedidæ and Trinucleidæ are homologous with the eye-lines of the trilobites with compound eyes, and that the ocelli on the cheeks are therefore degenerate compound eyes.
The simplest form of the genal cæcum is seen in the blind Elyx (Lindstroem 1901, pl. 6, fig. 43). The main trunk is at nearly right angles to the axis, the increase in its width is gradual in approaching the glabella, and an equal number of branches diverge from both sides.
Ptychoparia striata (Barrande 1852, pl. 14, figs. 1, 3) is an excellent example of a trilobite with compound eyes and genal cæca. It will be noted that the main trunk and the eye-line are coincident, and that both on the free and fixed cheeks the branches are all on the anterior side of the eye-line. Compare this with the condition in Conocoryphe (Barrande, pl. 14, fig. 8; Lindstroem, pl. 6, fig. 44), and one sees there a main branch having the same direction as in Ptychoparia and likewise with all the branches on the anterior side. At first sight this would seem to support the contention that these lines do lead out to the eyes, since Conocoryphe is blind, and the main trunk leads practically to the margin. But although Conocoryphe is blind, it has free cheeks, and the main trunk does not lead to the point on those free cheeks where eyes are to be expected, but back into the genal angles. And this direction holds in such diverse genera (as to eyes and free cheeks) as Harpes, Cryptolithus, Dionide, and Endymionia. In all these the genal cæca fade out in the genal angles, and in none of them would compound eyes be expected in that region. The coincidence of the eye-lines with the trunks of the genal cæca in Ptychoparia seems to be merely a coincidence. That the markings which radiate from the eyes of Ptychoparia and Solenopleura are not impressions made by nerves is obvious. That they are of the same nature as the similar markings in the eyeless trilobites is equally obvious. Ergo, they can not be nerves in either case, and that they have anything to do with the eyes is highly improbable. The eye was merely superimposed upon these structures.
The relation of the genal cæca to the ocelli on the cheeks is best shown in the Trinucleidæ. In all species of Tretaspis simple eyes are present, and in most of them there are very narrow eye-lines. The latter are occasionally continued beyond the ocular tubercle back to the genal angle. A similar course is seen in Harpes. If the simple eye is the homologue [84] of the compound eye, and the eye-line here the homologue of the eye-line in Ptychoparia, why does it continue beyond the eye? In any case, it can not be interpreted as a nerve. Cryptolithus tessellatus, when the cephalon is 0.45 mm. to 0.65 mm. long, shows short eye-lines and a small simple eye on each cheek. In some half-grown specimens, traces of the ocelli can be seen, but the eye-lines are absent. In the adult, both the eye-lines and the ocelli are entirely wanting. Reed states that "nervures" are also absent, and so they are from most specimens, but well preserved casts of the interior from the Upper Trenton opposite Cincinnati show them, and one cheek is here figured (fig. 25). As apparent from the figure, the main trunk is very short and gives rise to two principal branches, the first of which in its turn sends off lines from the anterior side. It was a specimen showing these lines which Ruedemann (1916, p. 147) figured as showing facial sutures. The interest lies in the fact that while the ocelli and eye-lines were lost in development, the genal cæca are present in the adult, showing that they are different structures.
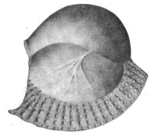
Fig. 25.—Cryptolithus tessellatus Green. Side view of the cheek of a specimen from the top of the Trenton opposite Cincinnati, Ohio, to show the branching genal cæca. These are the "facial sutures" of Ruedemann.
Harpides is another genus in which genal cæca are strikingly shown, and in this case they completely cover the huge cheeks, radiating from two main trunks to the front and sides. I have seen no good specimens, but it would appear from Angelin's figure (1854, pl. 41, fig. 7) that the rather large, simple eyes are not situated exactly on the vascular trunks. In the Harpides from Bohemia, the main trunks extend out with many branches beyond the simple eyes. It should be stated that the courses of the genal cæca are not correctly figured by Barrande (Supplement, 1872, pl. 1, fig. 11), as shown by casts of the original specimen in the Museum of Comparative Zoology. From Barrande's figure, one would suppose that the eye-lines and their continuation beyond the "ocelli" were superimposed upon the genal cæca without having any definite connection with them, but as a matter of fact the radial markings really diverge from the main trunks as in Elyx and similar forms.
As Reed has said, these lines are not mere ornamentation, but rather represent traces of structures of some functional importance. They probably can not be explained as traces of nerves and more likely represent either traces of the gastric cæca or of the circulatory [85] system. While they are known chiefly in Cambrian and Lower Ordovician trilobites, there is no evidence that the organs represented were not present in later forms, even if the shell may not have been affected by them. While they indicate very fine, thread-like canals, the present evidence seems to be in favor of assigning to them the function of lodging the glands which secreted the principal digestive fluids.
Volborth (1863, pl. 1, fig. 12 = our fig. 26) has described the only organ in a trilobite which suggests a heart. A Russian specimen of Illænus with the shell removed shows a somewhat flattened, tubular, chambered organ extending from under the posterior end of the cephalon to the anterior end of the pygidium. The posterior nine chambers were each 1.5 mm. long and 1.5 mm. wide, while the two anterior chambers were respectively 2.5 mm. and 3 mm. wide. These were all under the thorax, and at least two more chambers are shown under the cephalon, but rather obscurely. The species of the Illænus is not stated, but since no Illænus has more than ten segments in the thorax, and this tube has at least thirteen chambers, it is evident that its constrictions are inherent in it, and are not due to the segmentation of the thorax. Beecher has made a passing allusion to this organ as an alimentary canal. This was the original opinion of Volborth. Pander, however, suggested to him that it might be a heart. The alimentary canal of Cryptolithus does not show any constrictions, while the heart of Apus (see fig. 27) and other branchiopods does show them. It should be noted, further, that while this heart enlarges toward the front, it is everywhere very small as compared with the width of the axial lobe, and much narrower than sections of Ceraurus and Calymene would lead one to expect the alimentary canal of Illænus to be. Where the heart is 1.5 mm. to 3 mm. wide, the axial lobe is 11 mm. wide.
![]() |
![]() |
Fig. 26. Copy of Volborth's figure of the heart of Illænus. |
Fig. 27. Heart of Apus. Copied from Gerstäcker. |
While this may be merely a cast of the alimentary canal it is sufficiently like a heart to deserve consideration as such an organ.
Nothing suggesting a heart has been seen in the sections of Ceraurus and Calymene. The mesenteron and its sheath crowd so closely against the dorsal test in the anterior part [86] of the thorax that there seems to be no room for the heart, but it must have been located beneath the sheath and above the alimentary canal. If the latter were filled with mud, and the animals lay on their backs, as most of them did at death, the canal would drop down into the axial lobe and the soft heart would naturally disappear and leave 110 trace of its presence in the fossils.
The Median "Ocellus" or "Dorsal Organ."
Many trilobites, otherwise smooth, bear on the glabella a median pustule which is usually referred to as a simple eye or median ocellus, but whose function can not be said to have been certainly demonstrated. Ruedemann (1916, p. 127), who has recently made a careful study of this problem, lists about thirty genera, members of ten families, Agnostidæ, Eodiscidæ Trinucleidæ, Harpedidæ, Remopleuridæ, Asaphidæ Illænidæ, Goldiidæ, Cheiruridæ, and Phacopidæ, in which this tubercle is present, and had he wished he might have cited more, for it is of almost universal occurrence in Ordovician trilobites.
I have not especially searched the literature for references to this median tubercle. It is often mentioned by writers in descriptions of species, but apparently few have tried to explain it. Beyrich (1846, p. 30) suggested that it indicated the beginning of the alimentary canal. Barrande mentioned it, but if he gave any explanation, it has escaped me. McCoy (Syn. Pal. Foss. 1856, p. 146) called it an ocular (?) tubercle, and that seems to have been the interpretation which most writers on trilobites have assigned to it, if they suggested any function at all. Beecher (1895 B, p. 309) concurred in this opinion.
Bernard (1894, p. 422) ascribed to this tubercle, as well as to the median tubercle on the nuchal segment, an excretory function, comparing it with the "dorsal organ" in Apus.
Reed (1916, p. 174) states that it may be either the representative of the "dorsal" organ of the branchiopods, or a median unpaired ocellus.
Ruedemann (1916) has made the only real investigation of the subject. He came to the conclusion that it was a parietal eye, without a crystalline lens, but corresponding to the "parietal eye of other crustaceans, and especially of the phyllopods, which is a lens-shaped or pear-shaped sac, usually filled with sea water." He found that above the "ocellus" the test was usually thin or even absent, and in a few cases a dark line beneath seemed to outline the original form of the sac. His summary follows:
It is claimed that most, if not all, trilobites possessed a median or parietal eye on the glabella. [In proof of this assertion the following facts are stated:]
1. A great number of species, belonging to more than thirty genera, possess a distinct tubercle on the glabella. This tubercle occurs alone in many genera, otherwise smooth, as in the Asaphidæ, and is hence of functional importance.
2. In certain cases, as in Cryptolithus tessellatus, distinct lenticular bodies [not lenses] were recognized; in others, as in Asaphus expansus, only a thinner, probably transparent test. Many other species show a distinct pit in interior casts of the tubercle, indicating a lens-like thickening of the top of the tubercle. The median eye therefore probably possessed all the different stages of development seen in other crustaceans.
3. As in the parietal eyes of the crustaceans and the eurypterids, the tubercles are most prominent and distinct in the earlier growth-stages, notably so in Isotelus gigas.
4. The tubercle is especially well developed in the so called blind forms where the lateral eyes are abortive, as in Cryptolithus (Trinucleus), Dionide, Ampyx.
5. The tubercles always appear on the apex on the highest part of the glabella, where their visual function would be most useful.
6. The tubercle is generally situated between the lateral eyes, like the parietal eye in crustaceans and eurypterids, on account of its close connection with the brain.
7. Frequently it forms the posterior termination of a short crest, also as in certain eurypterids (Stylonurus), indicating the direction of the nerve.
8. The median eye is borne on a tubercle or mound in the Ordovician and Silurian trilobites, while the tubercle is rarely noticed in the Devonian and in few Cambrian forms. In the Devonian forms, similarly as in many crustaceans and in later growth-stages of some asaphids, the strong development of the lateral eyes may have led to a loss of the parietal eyes. In the Cambrian genera evidence is present to suggest that the parietal eyes consisted only of transparent spots or lens-like thickenings of the exoskeleton, hardly noticeable from the outside.
9. It is a priori to be inferred that the trilobites should, as primitive crustaceans, have possessed median or parietal eyes.
As a student, I accepted Professor Beecher's dictum that this tubercle represented a median ocellus, but more recently a number of things have led me to the view that it is the point of attachment of the ligament by which the heart is supported.
The chief arguments against its interpretation as a parietal eye seem to be that its structure is not absolute proof, being capable of other explanation; its position is variable, in front, between, or back of the eyes; it is exactly like other tubercles on the median line, especially the nuchal spine or tubercle, and the similar ones along the axial lobe of the thorax; and it is not present in the protaspis or very young trilobites.
1. The structure disclosed by Ruedemann's sections, a sort of sac-like cavity beneath a thinned test, can be explained as a gland, a ligamentary attachment, or a vestigial spine, as well as an eye. In a section of Asaphus expansus, which I made some years ago when trying to get some light on this problem, there is a similar cavity under the pustule, but a secondary layer of shell lay beneath it and apparently cut it off from the glabellar region, thus indicating that it had lost its function in the adult of this animal. Sections through the tubercles of the glabella of Ceraurus show all of them hollow, with very thin upper covering or none at all, and their structure is not unlike that of the tubercle of Cryptolithus. In fact, sections can be seen in Doctor Walcott's slices which are practically identical with the one Ruedemann obtained from Cryptolithus. Since it is obvious that not all of the pustules of a Ceraurus could have been eyes, the evidence from structure is rather against than for the interpretation of the median pustule as such an organ.
2. The position of the tubercle varies greatly in different genera. Where furthest forward (Tretaspis, Goldius), it is just back of the frontal lobe, while in some species of asaphids it is in the neck furrow. In species with compound eyes it is frequently between the eyes, but more often back of them. If its history be traced in a single family, it is generally found farthest forward in the more ancient species and moves backward in the more recent ones. The eyes do this same thing, but the median tubercle goes back further than the eyes. This can be seen, for example, in the American Asaphidæ, where the pustule is up between the eyes of Hemigyraspis and Symphysurus of the Beekmantown and back of the eyes of the Isotelus of the Trenton. Turning now to the under side of the head, it appears that the tubercle bears a rather definite relation to the hypostoma. If the hypostoma is short, the tubercle is well forward. If long, it is far back on the head. It seems in many cases to be just back of the posterior tip of the hypostoma, or just behind the position of the mouth, while in others it is not as far back as the tip of the hypostoma.
The median tubercle is in many cases developed into a long spine. This is usually in an ancient member of a tubercle-bearing family, and suggests that in most cases the tubercle is a vestigial organ. An example of this occurs in Trinucleoides, the most ancient of the Trinucleidæ. Trinucleoides reussi (Barrande) (Supplement, 1872, pl. 5, figs. 17, 18) has a very long slender spine in this position. It could be explained as an elevated median eye, but it also very strongly suggests the zoæal spine of modern brachyuran Crustacea. [88] Gurney (Quart. Jour. Mic. Sci., vol. 46, 1902, p. 462) supports Weldon in the conclusion that the long spines of the zoæa are directive, and states that the animal swims in the direction of the long axis of the spine. He also suggests that, since the period of their presence corresponds to the period before the development of the "auditory" organs, the spines may perform the functions of balancing and orientation. It is generally admitted that the spine of the zoæa is also protective, and the obvious function, first pointed out by Spence Bate in 1859, is that it contains a ligament which helps suspend the heart, which lies beneath the spine. This latter function may have been that of the median tubercle in the trilobite. Such an explanation would account for the backward migration mentioned above, for as the stomach enlarged and the mouth moved backward on the ventral side, the heart may have been pushed backward on the upper side.
There is also a curious parallelism between the ontogenetic history of the zoæal spine and the phylogenetic history of the Trinucleidæ or Cheiruridæ (Nieszkowskia is the ancient member of this family in which the spine replaces the tubercle). When first hatched, the larval crab shows no trace of the spine, but very quickly it evaginates, lying dorsally on the median line, pointing forward (Faxon, Bull. Mus. Comp. Zool., vol. 6, 1880, pl. 2). With the splitting of the original envelope, the spine becomes erect, but persists only a short time, and is reduced to a vestigial tubercle toward the end of the zoæal stages, its disappearance being, as pointed out by Gurney, coincident with the development of the balancing organs. This manner of suspension of the heart by a long tendon certainly does suggest that Gurney is right in his interpretation of the function. Briefly, the zoæal spine served for a short time a function later taken over by other organs. It was not present in the youngest stages, it became prominent at a very early stage, was soon vestigial, and then lost.
Take now the trilobites. There is no trace of the median pustule in the protaspis of any form, and in many primitive trilobites it is absent. It appears first as a long spine in certain families, and later becomes vestigial and disappears. Very few trilobites of Silurian and later times show it at all.
In the particular case of the Trinucleidæ, which were burrowers, the spine is present on only the oldest and most primitive of the group, a form which has only a most rudimentary fringe. It is obvious from the large size of the pygidium in the larval trinucleid that this family is derived from a group of free swimmers. Trinucleoides reussi was perhaps in the transitional stage, just leaving the swimming mode of life, and belonged to a group which had not developed any other "statocyst" than the median spine. Among the later Trinucleidæ the spine became a vestigial tubercle, and in some cases entirely disappeared. A similar history can be traced in the Cheiruridæ, starting from some such forms as the American Lower Ordovician Nieszkowskia (N. perforator p. ex.).
Another example of a median spine instead of a tubercle is in Goldius rhinoceros (Barrande). Since this species is not from the oldest Goldius-bearing rocks, but from the Lower Devonian, it does not follow what seems to be the general rule, but makes an interesting exception. Goldius rhinoceros (Barrande) (Supplement, 1872, pl. 9, figs. 12, 13) has the median tubercle elevated into a stubby, recurved spine very suggestive of the horn of a rhinoceros. Since the eyes of this species are very well developed, there seems no especial reason for the elevation of a parietal eye, and the example certainly does not support that interpretation.
3. This tubercle is essentially similar to other tubercles on the median line of cephalon, thorax, and even pygidium. This has been discussed sufficiently under section 1 above, but [89] it may perhaps be justifiable to point out that in some of the Agnostidæ there is a median tubercle on both shields, and since it has not yet been demonstrated beyond question which shield is the cephalon, to say which one is a parietal eye and which one is a tubercle is impossible. In other words, the parietal eye can not be differentiated from any other tubercle except by its position.
4. One of the as yet unexplained features of the protaspis of trilobites is the absence of the "nauplius eye." Beecher (1897 B, p. 40) explained this on the ground of the extremely small size of the protaspis and the imperfection of the preservation. If the median tubercle were really a median eye, it should be present in the protaspis and the earlier stages of the ontogeny, even if not in the adult, and should certainly appear before the compound eyes. (In Limulus, however, the compound eyes appear first.) The median eye has not so far been seen in any young trilobite in any stage previous to that in which compound eyes are present. The full ontogeny is not known of any species with compound eyes in which the median tubercle is present in the adult, but theoretically the median eye should be most prominent in the young of just those primitive trilobites about whose development most is known.
There has been a rather general impression among students of trilobites that the eye-lines, which should be differentiated from the genal cæca, denote the course of the optic nerves, but no other evidence of the nervous system has been found, save the so called nervures which have been discussed above. In Apus the nerves leading to the eyes come off from the anterior ganglion or "brain" and run directly to the eyes. If conditions were similar in the trilobites, the "brain" was beneath the anterior glabellar lobe, provided, of course, that the eye-lines do indicate the course of the optic nerve.
The ontogenetic history of the eye-lines of trilobites with compound eyes is instructive, and has already been discussed by Lindstroem (1901, pp. 12-25), but he did not cite the case of Ptychoparia, which is particularly interesting, because in this genus both eye-lines and "nervures" are present. Beecher (1895 C, p. 171, pl. 8, figs. 5-7) has shown that in Ptychoparia kingi the eye-lines of a specimen in the metaprotaspis stage run forward at a low angle with the glabella, while in the adult their course is nearly at right angles to it. They have therefore swung through an arc of at least 60 and in so doing have had ample opportunity to become coincident with the primary trunks of the genal cæca. Once that was accomplished, it is quite likely that the one fold in the shell would continue to house both structures. In other trilobites, there is a similar backward progression of the eye-lines.
As would be expected, the ventral ganglia and the longitudinal cords left no trace in the test. Since each segment has appendages, there was probably a continuous chain of ganglia back to the posterior end of the pygidium.
Dermal glands.—The surface of many trilobites is "ornamented" with pustules and spines which on sectioning are nearly always found to be hollow, and in many cases have a fine opening at the tip. While it is generally believed that the purpose of these spines was protective, yet it is possible that many of them were merely outgrowths which increased the area through which the respiratory function could be carried on. It will be recalled [90] that most of the smooth trilobites are punctate, some of them very conspicuously so, and the spines and pustules of ornamented trilobites may merely subserve the same function as the pores of smooth ones.
If the spines were protective, it would not be surprising if some of them, hollow and open at the top, were poisonous also, and had glands at the base. These are, however, purely matters of speculation so far.
Renal excretory organs.—Nothing has been seen of any such organs, unless the genal cæca may possibly be of that nature. The main trunks of these always lead to the sides of the anterior glabellar lobe, which is not the point of attachment of either antennæ or biramous limbs, so that there seems little chance that they will bear this interpretation.
Reproductive organs.—Nothing is yet positively known about the reproductive organs or the position of their external openings. If the "exites" of Neolenus could be interpreted as brood-pouches, which does not seem probable, then the genital openings were located near the base of some pair of anterior thoracic appendages.
The Panderian Organs: Internal Gills or Poison Glands?
At a meeting of the Mineralogical Society at St. Petersburg, Volborth (1857) announced that Doctor Pander had two years before discovered certain organs on the lower side of the doublure of the pleural lobes of the thorax of a specimen of Asaphus expansus. These organs were oval openings in the doublure, one near the posterior margin of the cephalon, and one on each thoracic segment of the half-specimen figured by Volborth in 1863. They were explained by Volborth and by Eichwald (1860, 1863) as the points of attachment of appendages. Billings (1870) described and figured the "Panderian organs" of "Asaphus platycephalus" and stated that he had seen them in Asaphus [Ogygites] canadensis and A. megistos [Isotelus maximus] as well. He thought some sort of organ was attached to them, but could not suggest its function. Woodward (1870) thought that the openings were "only the fulcral points on which the pleuræ move." Their position outside the fulcra shows that this explanation is impossible.
So far as I am aware, the Panderian organs have been seen only in the Asaphidæ. Barrande figured them in "Ogygia" [Hemigyraspis] desiderata (1872) and Schmidt in two species of Pseudasaphus. They seem to occupy the same position in Bohemian, Russian, and American specimens. There is always one pair of openings on each thoracic segment, and one pair in line with them on the posterior margin of the cephalon. They occur near the anterior margin of the segment, and near the inner end of the doublure. In some cases they are surrounded by a ventrally projecting rim, while in others they have a thin edge. There seem to be no markings on the interior of the shell which are connected with them.
While thinking over the trilobites in connection with the origin of insects, it occurred to me that these hitherto unexplained Panderian organs might possibly be openings to internal gills and that the Asaphidæ might have been tending toward an amphibious existence. On mentioning this to Doctor R. V. Chamberlin of the Museum of Comparative Zoology, he called my attention to the possibility that they might be openings similar to those of the repugnatorial glands of Diplopoda. While no definite decision as to the function can be made, the explanation offered by Doctor Chamberlain seems more plausible than my own, and has suggested still a third, namely, that they might be the openings of poison glands.
If one were to argue that these apertures are really connected with respiration, it might be pointed out that they are ventral in position, while the foramina repugnatoria are always [91] dorsal or lateral, even in diplopods with broad lateral expansions. If offensive secretions were poured out beneath a concave shell like that of a trilobite, they would be so confined as to be but slightly effective against an enemy. This would indicate that if these openings were the outlets of glands, the substance secreted might be a poison used to render prey helpless. On the other hand, openings to gills are normally ventral in position, and if the pleural lobes were folded down against the body, they would be brought very close to the bases of the legs.
A further curious circumstance is that so far no traces of exopodites have been found on Isotelus. The endopodites of both Isotelus latus and I. maximus are fairly well preserved in the single known specimen of each, yet no authentic traces of exopodites have been found with them. Moreover, Walcott sliced specimens of Isotelus from Trenton Falls and found only endopodites. It may also be recalled that the finding of the specimen of Isotelus arenicola at Britannia and the tracks which I attributed to it, suggested to me that it was a shore-loving animal (1910). It offers a field for further inquiry, whether the Asaphidæ may not have had internal gills, and whether some primitive member of the family may not have given rise to tracheate arthropods.

Fig. 28. Side view of a specimen of Isotelus gigas Dekay, from which the test of the pleural lobes has been broken to show the position of the Panderian organs. Natural size. Specimen in the Museum of Comparative Zoology.
The explanation of the Panderian organs as openings of poison glands is less radical than the one just set forth, and so possibly lies nearer the truth. One would expect poison glands to lie at the bases of fangs, and so they do in specialized animals like chilopods and scorpions, but the trilobites may have had the less effective method of pouring out the poison from numerous glands. The purpose may have been merely to paralyze the brachiopod or pelecypod which was incautious enough to open its shell in proximity to the asaphid.
This is a field which is rather one for investigation than for exposition. Very little has been done, though probably much could be. The chief obstacle to a clearer understanding of the muscular system lies in the difficulty of getting at the inner surface of the test without obscuring the faint impressions in the process.
There exist in the literature a number of references to scars of attachment of muscles, and any study of the subject should of course begin by the collection of such data. I shall at this time refer to only a few observations on the subject.
The structure and known habits of trilobites make it obvious that strong flexor and extensor muscles must have been present, and some trace of them and of their points of attachment should be found. It is likely that their proximal ends were tough tendons. The muscles holding up the heart and alimentary canal would be less likely to reveal their presence [92] by scars, but there must have been at least one pair of strong muscles extending from the under side of the head across to the hypostoma. Judging from the method of attachment, the muscles moving the limbs were short ones, chiefly within the segments of the legs themselves.
Since the majority of trilobites had the power of enrollment, and seem also to have used the pygidia in swimming, the flexors must have been important muscles. Beecher (1902, p. 170) appears to have been the only writer to point out any tangible evidence of their former presence. Walcott (1881, p. 199) had shown that the ventral membrane was reinforced in each segment by a slightly thickened transverse arch. Beecher showed that on this thickened arch in Triarthrus, Isotelus, Ptychoparia, and Calymene, there are low longitudinal internal ridges or folds. One of these is central, and there is a pair of diagonal ridges on either side. Beecher interpreted these ridges as separating the strands of the flexor muscles, and believed that a line of median ridges divided a pair of longitudinal muscles, while the outer ridges showed the place of attachment of the pair of strands which was set off to each segment. He did not discuss the question as to where the anterior and posterior ends were attached. In trilobites with short pygidia, the attachment would probably have been near the posterior end, and it is possible that the two scars beneath the doublure and back of the last appendifers in Ceraurus may indicate the point of attachment in that genus.
There is as yet no satisfactory evidence as to where the anterior ends of the flexors were attached. In Apus these muscles unite in an entosternal sinewy mass above the mouth, but no evidence of any similar mass has been found in the trilobites and it is likely that the muscles were anchored somewhere on the test of the head.
The exact position of these muscles has not been previously discussed. The interior of the dorsal test shows no such apodemes as are found on the mesosternites, but, as I have shown in the discussion of the alimentary canal of Calymene and Ceraurus, there is an opening on either side of the axial lobe between the dorsal test and the abdominal sheath, and it is entirely probable that an extensor muscle passed through each of these. The abdominal sheath extends only along the posterior region of the glabella and the anterior part of the thorax, and probably served to protect the soft organs from the strain of the heavy muscles. The extensors (see fig. 29) probably lay along the top of the axial lobe on either side of the median line of the thorax to the pygidium, where they appear to have been attached chiefly on the under side of the anterior ring of the axial lobe, although strands probably continued further back. This is above and slightly in front of the fulcral points on the pleura, and meets the mechanical requirements. Ceraurus (Walcott, 1875, and 1881, p. 222, pl. 4, fig. 5) shows a pair of very distinct scars on the under side of the first ring of the pygidium, and in many other trilobites (Illænus, Goldius, etc.) distinct traces of muscular attachment can be seen in this region, even from the exterior. The anterior ends were probably attached by numerous small strands to the top of the glabella, and, principally, to the neck-ring.
On enrolling, the sternites of all segments are pulled forward and the tergites backward. In straightening out, the reverse process takes place. The areas available for muscular attachment [93] are so disposed as to indicate longitudinal flexor and extensor muscles rather than short muscles extending from segment to segment. Indeed, the tenuity of the ventral membrane is such as to preclude the possibility of enrollment by the use of muscles of that sort, while powerful longitudinal flexors could have been anchored to cephalon and pygidium. The strongly marked character of the neck-ring of trilobites is probably to be explained as due to the attachment of the extensor muscles, rather than to its recent incorporation in the cephalon. The same is true of the anterior ring on the pygidium.
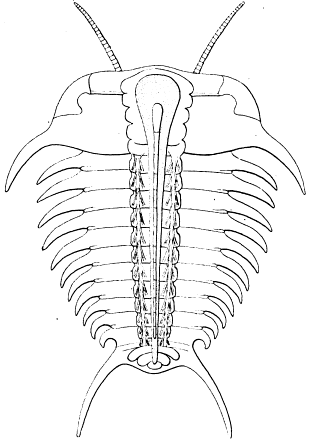
Fig. 29. Restoration of a part of the internal organs of Ceraurus pleurexanthemus as seen from above. At the sides are the extensor muscles, and in the middle, the heart overlying the alimentary canal. Drawn by Doctor Elvira Wood, under the supervision of the author.
Possible preservations of extensors and flexors in Ceraurus.—Among Doctor Walcott's sections are four slices which I should not like to use in proving the presence of longitudinal muscles, but which may be admitted as corroborative evidence. Two of these transverse sections (Nos. 114 and 199) show a dorsal and a ventral pair of dark spots in positions which suggest that they represent the location of the dorsal and ventral muscles, while two others (Nos. 131 and 140) show only the upper pair of spots. The chief objection to this [94] interpretation is that it is difficult to imagine how the muscles could be so replaced that they happen to show in the section. Both the sections showing all four spots are evidently from the anterior part of the thorax, as they show traces of the abdominal sheath, which seems to be squeezed against the inside of the axial lobe, with the muscles (?) forced out to the sides. The ventral pair lie just inside the appendifers, but even if they are sections of muscles, all four are probably somewhat out of place.
The hypostoma fits tightly against the epistoma, or the doublure when the epistoma is absent, but in no trilobite has it ever been seen ankylosed to the dorsal test, and its rather frail connection therewith is evidenced by the relative rarity of specimens found with it in position. That the hypostoma was movable seems very probable, and that it was held in place by muscles, certain. The maculæ were always believed to be muscle scars until Lindstroem (1901, p. 8) announced the discovery by Liljevall of small granules on those of Goldius polyactin (Angelin). These were interpreted as lenses of eyes by Lindstroem, who tried to show that the maculæ of all trilobites were functional or degenerate eyes. Most palæontologists have not accepted this explanation, and since the so called eyes cover only a part of the surface of the maculæ, it is still possible to consider the latter as chiefly muscle-scars.
In Lindstroem's summary (1901, pp. 71, 72) it is admitted that the globular lenses are found only in Bronteus (Goldius) (three Swedish species only) and Cheirurus spinulosus Nieszkowski, while the prismatic structure supposed to represent degenerate eyes was found in eleven genera (Asaphidæ, Illænidæ, Lichadidæ). All of these are forms with well developed eyes, and Lindstroem himself points out that the appearance of actual lenses in the hypostoma was a late development, long after the necessity for them would appear to have passed.
The use of the hypostoma has been discussed by Bernard (1892, p. 240) and extracts from his remarks are quoted:
The earliest crustacean-annelids possessed large labra or prostomia projecting backward, still retained in the Apodidæ and trilobites. This labrum almost necessitated a very deliberate manner of browsing. The animal would creep along, and would have to run some way over its food before it could get it into its mouth, the whole process, it seems to us, necessitating a number of small movements backwards and forwards. Small living prey would very often escape, owing to the fact that the animal's mouth and jaws were not ready in position for them when first perceived. The labrum necessitates the animal passing forwards over its prey, then darting backward to follow it with its jaws. We here see how useful the gnathobases of Apus must be in catching and holding prey which had been thus passed over. Indeed the whole arrangement of the limbs of Apus with the sensory endites forms an excellent trap to catch prey over which the labrum has passed.
In alcoholic specimens of Apus the labrum is not in a horizontal plane, as it is in most well preserved trilobites, but is tipped down at an angle of from 30 to 45, and the big mandibles lie under it. It has considerable freedom of motion and is held in place by muscles which run forward and join the under side of the head near its posterior margin. It seems entirely possible that the hypostoma of the trilobite had as much mobility as the labrum of Apus, and that by opening downward it brought the mouth lower and nearer the food. It will be recalled that the hypostomata of practically all trilobites are pointed at the posterior margin, there being either a central point or a pair of prongs. By dropping down the hypostoma until the point or prongs rested on or in the substratum, and sending food forward [95] to the mouth by means of the appendages, a trilobite could make of itself a most excellent trap, and if the animal could dart backward as well as forward, the hypostoma would be still more useful. There is no reason to suppose that they could not move backward, and the "pygidial antennæ" of Neolenus indicate that animals of that genus at least did so. This habit of dropping down the hypostoma would also permit the use of those anterior gnathobases which seem too far ahead of the mouth in the trilobites with a long hypostoma.
For actual evidence on this point, it is necessary to have recourse once more to Doctor Walcott's exceedingly valuable slices. From such sections of Ceraurus as his Nos. 100, 106, 108, 170, and 173, it is evident that the hypostoma of that form could be dropped considerably without disrupting the ventral membrane (fig. 30). Sections of Calymene already published (Walcott 1881, pl. 5, figs. 1, 2) show the hypostoma turned somewhat downward, and the slices themselves show sections of the anterior pair of gnathobases beneath the hypostoma. When the hypostoma was horizontal, these gnathobases were crowded out at the sides.
If the hypostoma were used in the manner indicated, the muscles must have been more efficient than those of the labrum of Apus, and it is probable that they crossed to the dorsal test. Just where they were attached is an unsolved problem. Barrande (1852, pl. 1, fig. 1) has indicated an anterior pair of scars and a single median one on the frontal lobe of Dalmanites that may be considered in this connection, and also three pairs of scars on the last two lobes of the glabella of Proëtus (1852, pl. 1, fig. 7). Moberg (1902, p. 295, pl. 3, figs. 2, 3, text fig. 1) has described in some detail the muscle-scars of a rather remarkable specimen of Nileus armadillo Dalman. While, as I shall point out, I do not agree wholly with Professor Moberg's interpretation, I give here a translation (made for Professor Beecher) of his description, with a copy of his text figure:
The well preserved surface of the shell permits one to note not only the tubercle (t) but a number of symmetrically arranged glabellar impressions. And because of their resemblance to the muscular insertions of recent crustaceans, I must interpret them as such. They appear partly as rounded hollows (k and i), also as elongate straight or curved areas (a, b, c, e, g, h) made up of shallow impressions or furrows about 1 mm. long, sub-parallel, and standing at an angle to the trend of the areas. Impression e is especially well [96] marked, inasmuch as the perpendicular furrows are arranged in a shallow crescentic depression; and impression d shows besides the obscure furrows a number of irregularly rounded depressions. Larger similar ones occur at f, and in part extend over toward g.
The meaning of these impressions, or their myologic significance, could be discussed, although such discussion might rather be termed guessing.
Inner organs, such as the heart and stomach, might have been attached to the shell along impressions a and b. Also along or behind c and h, which both continue into the free cheeks, ligaments or muscular fibers may have been inserted. From d, e, f, and g, muscles have very likely gone out to the cephalic appendages. Against this it may be urged that impression d is too far forward to have belonged to the first pair of feet. Again, the impression h may in reality represent two confluent muscular insertions, from the first of which, in that case, arose the muscles of the fourth pair of cephalic feet. Were this the case, the muscles of the first pair of cheek feet should be attached at e. And d in turn may be explained as the attachment of the muscles of the antennæ, k those of the hypostoma, and from i possibly those of the epistoma. That k is here named as the starting point of the hypostomial muscles and not those of the antennæ, depends partly on the analogous position of i and partly on the fact that the hypostoma of Nileus armadillo (text figure, in which the outline of the hypostoma is dotted), by reason of it? wing-like border, could not have permitted the antennæ to reach forward, but rather only outward or backward.
My own explanation would be that impressions e, f, and g correspond to the glabellar furrows, h the neck furrow, and all four show the places of attachment of the appendifers. Those at d may possibly be connected with the antennæ, although I should expect those organs to be attached under the dorsal furrows at the sides of the hypostoma. It will be noted that either b, k, or i correspond well with the maculæ of the hypostoma and some or all of them may be the points of attachment of hypostomial muscles. They correspond also with the anterior scars of Dalmanites.
While I have nothing to add to what has been written about the eyes of trilobites, this sketch of the anatomy would be incomplete without some reference to the little which has been done on the structure of these organs.
Quenstedt (1837, p. 339) appears to have been the first to compare the eyes of trilobites with those of other Crustacea. Johannes Müller had pointed out in 1829 (Meckel's Archiv) that two kinds of eyes were found in the latter group, compound eyes with a smooth cornea, and compound eyes with a facetted coat. Quenstedt cited Trilobites esmarkii Schlotheim (=Illænus crassicauda Dalman) as an example of the first group, and Calymene macrophthalma Brongniart (=Phacops latifrons Bronn) for the second. Misreading the somewhat careless style of Quenstedt, Barrande (1852, p. 133) reverses these, one of the few slips to be found in the voluminous writings of that remarkable savant.
Burmeister (1843; 1846, p. 19) considered the two kinds of eyes as essentially the same, and accounted for the conspicuous lenses of Phacops on the supposition that the cornea was thinner in that genus than in the trilobites with smooth eyes.
Barrande (1852, p. 135) recognized three types of eyes in trilobites, adding to Quenstedt's smooth and facetted compound eyes the groups of simple eyes found in Harpes. In his sections of 1852, pl. 3, figs. 15-25, which are evidently diagrammatic, he shows separated biconvex lenses in both types of compound eyes, Phacops and Dalmanites on one hand, and Asaphus, Goldius, Acidaspis, and Cyclopyge on the other. Clarke ( 1888), Exner ( 1891 ) and especially Lindstroem (1901) have since published much more accurate figures and descriptions. The first person to study the eye in thin section seems to have been Packard (1880), who published some very sketchy figures of specimens loaned him by Walcott. He [97] studied the eyes of Isotelus gigas, Bathyurus longispinus, Calymene, and Phacops, and decided that the two types of eyes were fundamentally the same. He also compared them with the eyes of Limulus.
Clarke (1888), in a careful study of the eye of Phacops rana, found that the lenses were unequally biconvex, the curvature greater on the inner surface. The lens had a circular opening on the inner side, leading into a small pear-shaped cavity. The individual lenses were quite distinct from one another, and separated by a continuation of the test of the cheek.
Exner (1891, p. 34), in a comparison of the eyes of Phacops and Limulus, came to the opinion that they were very unlike, and that the former were really aggregates of simple eyes.
Lindstroem (1901, pp. 27-31) came to the conclusion that besides the blind trilobites there were trilobites with two kinds of compound eyes, trilobites with aggregate eyes, and trilobites with stemmata and ocelli. His views may be briefly summarized.
I. Compound eyes.
1. Eyes with prismatic, plano-convex lenses.
"A pellucid, smooth and glossy integument, a direct continuation of the common test of the body, covers the corneal lenses, quite as is the case in so many of the recent Crustacea. The lenses are closely packed, minute, usually hexagonal in outline, flat on the outer and convex on the inner surface. Such eyes are best developed in Asaphus, Illænus, Nileus, Bumastus, Proëtus, etc."
2. Eyes with biconvex lenses.
The surface of the eye is a mass of contiguous lenses, covered by a thin membrane which is frequently absent from the specimens, due to poor preservation. The lenses are biconvex, and being in contact with one another, are usually hexagonal, although in some cases they nearly retain their globular shape. Such eyes are found in Bury care, Peltura, Sphæropthalmus, Ctenopyge, Goldius, Cheirurus, and probably others.
II. Aggregate eyes.
The individual lenses are comparatively large, distinct from one another, each lying in its own socket. There is, however, a thin membrane, which covers all those in any one aggregate, and is a continuation of the general integument of the body. This membrane is continued as a thickened infolding which forms the sockets of the lenses.
Such eyes are known in the Phacopidæ only.
III. Stemmata and ocelli.
The stemmata are present only in Harpes, where there may be on the summit of the cheek two or three ocelli lying near one another. Each, viewed from above, is nearly circular in outline, almost hemispheric, glossy and shining. In section they prove to be convex above and flat or slightly concave beneath. The test covers and separates them, as in the case of the aggregate eyes.
The ocelli of the Trinucleidæ and Eoharpes are smaller, and the detailed structure not yet investigated.
Lindstroem concludes that so far as its facets or lenses are concerned, the eye of the trilobite shows the greatest analogy with the Isopoda, and the least with Limulus.
The simplest eyes found among the Trilobita are the ocelli. These consist of a Simple thickening of the test to form a convex surface capable of concentrating light. The similarity in position of the paired ocelli of trilobites and the simple eyes of copepods has perhaps a significance.
The schizochroal eyes may well be compared with the aggregate eyes of the chilopods and scorpions. The mere presence of a common external covering is not sufficient to prove this a true compound eye, especially as the covering is merely a continuation of the general test.
The holochroal eyes are of two kinds, one with plano-convex and one with biconvex lenses. The latter would seem to be mechanically the more perfect of the two, and it is worthy of note that the trilobites possessing the biconvex lenses have, in general, much smaller eyes than those with the other type.
If, as some investigators claim, the parietal eye of Crustacea originates by the fusion of two lateral ocelli, trilobites show a primitive condition in lacking this eye, which may have originated through the migration toward the median line of ocelli like those of the Trinucleidæ.
That the sexes were separate in the Trilobita there can be very little doubt, but the study of the appendages has as yet revealed nothing in the way of sexual differences. One of the most important points still to be determined is the location of the genital openings.
In many modern Crustacea, the antennæ or antennules are modified as claspers, and it is barely possible that the curious double curvature of the antennules of Triarthrus indicates a function of this sort. The antennules of many specimens have the rather formal double curvature, turning inward at the outer ends, and retain this position of the frontal appendages, no matter what may be the condition of those on the body. Other specimens have the antennules variously displaced, indicating that they are quite flexible. It is conceivable that the individuals with rigid antennules are males, the others females.
It is interesting to note that the antennules of Ptychoparia permulta Walcott (1918, pl. 21, fig. 1) have the same recurved form. All the specimens of Neolenus, however, show very flexible antennas.
Barrande and Salter laid great stress upon the "forme longue" and "forme large" as indicating male and female. This was based upon the supposition that the female of any animal would probably have a broader test than the male, a hypothesis which seems to be very little supported by fact. In practical application it was found that the apparent difference was so often due to the state of preservation or the confusion of two or more species, that for many years little reference has been made to this supposed sex difference.
In his classic work on the trilobites of Bohemia, Barrande described three kinds of spherical and one of capsule-shaped bodies which he considered to be the eggs of trilobites. After a review of the literature and a study of specimens in the collections of the Museum of Comparative Zoology, it can be said that none of these fossils has proved to be a trilobite egg, but that they may be plants. A full account of them will be published elsewhere.
Walcott (1881) and Billings (1870) have described similar bodies within the tests of Calymene and Ceraurus, but without showing positive evidence as to their nature.
This is a subject upon which much can be inferred, but little proved. Without trying to cover all possibilities, it may be profitable to see what can be deduced from what is known of the structure of the external test, the internal anatomy, and the appendages. This can, to a certain extent, be controlled by what is inferred from the strata in which the specimens are found, the state of preservation, and the associated animals. (For other details, see the discussion of "Function of the Appendages" in Part I.)
The methods of locomotion may be deduced with some safety from a study of the appendages, and, as has repeatedly been pointed out, all trilobites could probably swim by their use. This swimming was evidently done with the head directed forward, and could probably be accomplished indifferently well with either the dorsal (gastronectic, Dollo) or the ventral (notonectic) side up. If food were sought on the bottom by means of sight, the animal would probably swim dorsal side up, for by canting from side to side it could see the bottom just as easily as though it were ventral side up, and at the same time it would be in position to drop quickly on the prey. In collecting food at the surface, it might swim ventral side up.
All trilobites could probably crawl by the use of the appendages, and, as has already been pointed out, there are great differences in the adjustment of the appendages to different methods of crawling. Some crawled on their "toes," some by means of the entire endopodites, and some apparently used the coxopodites to push themselves along. That the normal direction of crawling was forward is indicated by the position of the eyes and sensory antennules. There is no evidence that their mechanism was irreversible, however, and the position of the mouth and the shape of the hypostoma indicate that they usually backed into feeding position. The caudal rami of Neolenus were evidently sensory, and the animal was prepared to go in either direction.
The use of the pygidium as a swimming organ, suggested by Spencer (1903, p. 492) on theoretical grounds, developed by Staff and Reck (1911, p. 141) from a mechanical standpoint, and elaborated in the present paper by evidence from the ontogeny, phylogeny, and musculature, provided the animal with a swifter means of locomotion. By a sudden flap of this large fin, a backward darting motion could be obtained, which would be invaluable as a means of escape from enemies. Staff and Reck seem to think that in this movement the two shields were clapped together, and that the animal was projected along with the hinge-like thorax forward. This might be a very plausible explanation in the case of the bivalve-like Agnostidæ, and it is one I had suggested tentatively for that family before I read Staff and Reck's paper. In the case of the large trilobites with more segments, however, it would be more natural to think of a mode of progression in which there was an undulatory movement of the body and the pygidium, up-and-down strokes being produced by alternately contracting the dorsal and ventral muscles. Bending the pygidium down would tend to pull the animal backward, while bringing it back into position would push it forward. It follows, therefore, that one of these movements must have been accomplished very quickly, the other slowly. If the muscle scars have been interpreted properly, the ventral muscles were probably the more powerful, an indication that the animal swam backward, using the cephalon and antennules as rudders.
The chief objection to the theory of swimming by clapping the valves together is that where the thorax consists of several segments it no longer acts like the hinge of a bivalve, and a sudden downward flap of the pygidium would impart a rotary motion to the animal. Take, for example, such nearly spherical animals as the Illænidæ, and it will readily be seen that there is nothing to give direction to the motion if the pygidium be brought suddenly against the lower surface of the cephalon. A lobster, it is true, progresses very well by this method, but it depends upon its great claws and long antennæ to direct its motions. The whole shape of the trilobite is of course awkward for a rapidly swimming animal. It could keep afloat with the minimum of effort and paddle itself about with ease, but it was not built on the correct lines for speed.
Dollo (1910, p. 406), and quickly following his lead, Staff and Reck (1911, p. 130), have published extremely suggestive papers, showing that by the use of the principle of correlation of parts, much can be inferred about the mode of life of the trilobites merely from the structure of the test.
Dollo studied the connection between the shape of the pygidium and the position and character of the eyes. As applied by him, and later by Clarke and Ruedemann, to the eurypterids, this method seems most satisfactory. He pointed out that in Eurypterida like Stylonurus and Eurypterus, where there is a long spine-like telson, the eyes are back from the margin, so that a Limulus-like habit of pushing the head into the sand by means of the limbs and telson was possible. Erettopterus and Pterygotus, on the other hand, have the eyes on the margin, so that the head could not be pushed into the mud without damage, and have a fin-like telson, suggesting a swimming mode of life.
In carrying this principle over to the trilobites, Dollo was quite successful, but Staff and Reck have pointed out some modifications of his results. The conclusions reached in both these papers are suggestive rather than final, for not all possible factors have been considered. The following are given as examples of interesting speculations along this line.
Homalonotus delphinocephalus, according to Dollo, was a crawling animal adapted to benthonic life in the euphotic region, and an occasional burrower in mud. This is shown by well developed eyes in the middle of the cephalon, a pointed pygidium, and the plow-like profile of the head. This was as far as Dollo went. From the very broad axial lobe of Homalonotus it is fair to infer that, like Isotelus, it had very long, strong coxopodites which it probably vised in locomotion, and also very well-developed longitudinal muscles, to be used in swimming. From the phylogeny of the group, it is known that the oldest homalonotids had broad unpointed pygidia of the swimming type, and that the later species of the genus (Devonian) are almost all found in sandstone and shale, and all have wider axial lobes than the Ordovician forms. It is also known that the epistoma is narrower and more firmly fused into the doublure in later than in earlier species. These lines of evidence tend to confirm Dollo's conclusion, but also indicate that the animals retained the ability to swim well.
On the same grounds, Olenellus thompsoni and Dalmanites limulurus were assigned the same habitat and habits. Both were considered to have used the terminal spine as does Limulus.
Olenellus thompsoni is generally considered to be unique among trilobites in having a Limulus-like telson in place of a pygidium. This "telson" has exactly the position and characteristics of the spine on the fifteenth segment of Mesonacis, and so long ago as 1896, Marr (Brit. Assoc. Adv. Sci., Rept. 66th Meeting, page 764) wrote:
The posterior segments of the remarkable trilobite Mesonacis vermontana are of a much more delicate character than the anterior ones, and the resemblance of the spine on the fifteenth "body segment" of this species to the terminal spine of Olenellus proper, suggests that in the latter subgenus posterior segments of a purely membranous character may have existed devoid of hard parts.
This prophecy was fulfilled by the discovery of the specimens which Walcott described as Pædeumias transitans, a species which is said by its author to be a "form otherwise identical with O. thompsoni, [but] has rudimentary thoracic segments and a Holmia-like pygidium posterior to the fifteenth spine-bearing segment of the thorax." A good specimen of this form was found at Georgia, Vermont, associated with the ordinary specimens of Olenellus thompsoni, and I believe that it is merely a complete specimen of that species. [101] Olenellus gilberti, which was formerly supposed to have a limuloid telson, has now been shown by Walcott (Smithson. Misc. Coll., vol. 64, 1916, p. 406, pl. 45, fig. 3) to be a Mesonacis and to have seven or eight thoracic segments and a small plate-like pygidium back of the spine-bearing fifteenth segment. All indications are that the spine was not in any sense a pygidium. Walcott states that Olenellus resulted from the resorption of the rudimentary segments of forms such as Mesonacis and Pædeumias, leaving the spine to function as a pygidium. This would mean the cutting off of the anus and the posterior part of the alimentary canal, and developing a new anal opening on the spine of one of the thoracic segments!
If the spine of the fifteenth segment is not a pygidium, could it be used, as Dollo postulates, as a pushing organ? Presumably not, for though in entire specimens of Olenellus (Pædeumias) it extends back beyond the pygidium, it probably was borne erect, like the similar spines in Elliptocephala, and not in the horizontal plane in which it is found in crushed specimens.
While this removes some of the force of Dollo's argument, his conclusion that Olenellus was a crawling, burrowing animal living in well lighted shallow waters was very likely correct. The long, annelid-like body indicates numerous crawling legs, there is no swimming pygidium, and the fusion of the cheeks in the head makes a stiff cephalon well adapted for burrowing.
Staff and Reck have pointed out that Dalmanites limulurus was not entirely a crawler, but, as shown by the large pygidium, a swimmer as well. This kind of trilobite probably represents the normal development of the group in Ordovician and later times. The Phacopidæ, Proëtidæ, Calymenidæ, and other trilobites of their structure could probably crawl or swim equally well, and could escape enemies by darting away or by "digging themselves in."
Cryptolithus tessellatus (Trinucleus concentricus) is cited by Dollo as an example of an adaptation to life in the aphotic benthos, permanently buried in the mud. In this case he appealed to Beecher's interpretation of the appendages, and pointed out that while the adult is blind, the young have simple eyes and probably passed part of their life in the lighted zone. It needs only a glance at the very young to convince one that the embryos had swimming habits, so that in this form one sees the adaptation of the individual during its history to all modes of life open to a trilobite. The habits of the Harpedidæ may have been similar to those of the Trinucleidæ, but the members of this family are supplied with broad flat genal spines. It has been suggested that these served like pontoons, runners, or snow-shoes, to enable the animal to progress over soft mud without sinking into it. Some such explanation might also be applied to the similar development in the wholly unrelated Bathyuridæ. The absence of compound eyes and the poor development of ocelli in the Harpedidæ suggest that they were burrowers, and from these two families, Trinucleidæ and Harpedidæ, it becomes evident that a pygidial point or spine is not a necessary part of the equipment of a burrowing trilobite. In fact, from the habits of Limulus it is known that the appendages are relied upon for digging, and that the telson is a useful but not indispensable pushing organ.
Deiphon is an interesting trilobite from many points of view. Its pleural lobes are reduced to a series of spines on either side of the body, and its pygidium is a mere spinose vestige. Dollo considered this animal a swimmer in the euphotic zone, because its eyes are on the anterior margin, its body depressed, its glabella globose, and its pygidium flat [102] and spinose. That such a method of life was secondary in a cheirurid was indicated to him by the fact that the more primitive members of the family seemed adapted for crawling. Staff and Reck have gone further and shown that the pygidium is only the vestige of a swimming pygidium, and that the great development of spines suggests a floating rather than a swimming mode of life. They therefore argue for a planktonic habitat. A similar explanation is suggested for Acidaspis and other highly spinose species.
The Aeglinidæ, or Cyclopygidæ as they are more properly called, present the most remarkable development of eyes among the trilobites. In this, Dollo saw, as indeed earlier writers have, an adaptation to a region of scanty light. The cephalon is not at all adapted to burrowing, but the pygidium is a good swimming organ, and one is apt to agree that this animal was normally an inhabitant of the ill lighted dysphotic region, but also a nocturnal prowler, making trips to the surface at night. Similar habits and habitat are certainly indicated for Telephus and the Remopleuridæ, but whether Nileus and the large-eyed Bumastus are capable of the same explanation is doubtful.
Finch (1904, p. 181) makes the suggestion that "Nileus" (Vogdesia) vigilans, an abundant trilobite in the calcareous shale of the Maquoketa, was in the habit of burying itself, posterior end first. He found a slab containing fifteen entire specimens, all of which had the cephalon extended horizontally near the surface of the stratum, and the thorax and pygidium projecting downward. The rock showed no evidence that they were in burrows, and the fact that all were in the same position indicates that the attitude was voluntarily assumed. They appear to have entrenched themselves by the use of the pygidia, which are incurved plates readily adapted for such use, and, buried up to the eyes, awaited the coming of prey, but were, apparently, smothered by a sudden influx of mud. The form of the eye in Vogdesia vigilans bears out this supposition of Finch's. Not only are the eyes unusually tall, but the palpebral lobe is much reduced, so that many of the lenses look upward and inward, as well as outward, forward and backward. The particular food required by V. vigilans must have been very plentiful in the Maquoketa seas of Illinois and Iowa, for the species was very abundant, but that its habits were self-destructive is also shown by the great number of complete enrolled specimens of all ages now found there. The soft mud was apparently fatal to the species before the end of the Maquoketa, for specimens are seen but very rarely in the higher beds.
Vogdesia vigilans is shaped much like Bumastus, Illænus, Asaphus, Onchometopus, and Brachyaspis, and it may be that these trilobites with incurved pygidia had all adopted the habit of digging in backward. As noted above, their pygidia are not very well adapted for swimming, and most of them have large or tall eyes.
Dollo's comparison of the Cyclopygidæ to the huge-eyed modern amphipod Cystosoma is instructive. This latter crustacean, which has the greater part of the dorsal surface of the carapace transformed into eyes, is said to live in the dysphotic zone, at depths of from 40 to 100 fathoms, and to come to the surface at night. It swims ventral side down.
The kinds of sediments in which trilobites are entombed have so far afforded little evidence as to their habitat. Frech (Lethæa palæozoica, 1897-1902, p. 67 et seq.) who has collected such evidence as is available on this subject, places as deeper water Ordovician deposits the "Trinucleus-Schiefer" of the upper Ordovician of northern Europe and Bohemia, the "Triarthrus-Schiefer" of America, the "Asaphus-Schiefer" of Scandinavia, Bohemia, Portugal, and France, and the Dalmania quartzite of Bohemia. .
Cryptolithus and Triarthrus, although not confined to such deposits, are apt to occur [103] chiefly in very fine-grained shales, in company with graptolites. These latter are distributed by currents over great distances within short periods. It is somewhat curious that the nearly blind burrowing Trinucleidæ, the dysphotic, large-eyed Remopleuridæ and Telephus, the blind nektonic Agnostidæ and Dionide, and the planktonic graptolites should go together and make up almost the entire fauna of certain formations. Yet, when the life history of each type is studied, a logical explanation is readily at hand, for all have free-swimming larvæ.
A list of the methods of life noted above is given by way of summary, with examples.
Pelagic | ![]() |
Planktonic | Primarily Secondarily |
Earliest protaspis of all trilobites Deiphon, Odontopleura, etc. |
||||
Nektonic | ![]() |
Primarily | Later protaspis of all trilobites. | |||||
Secondarily | ![]() |
Probably many thin-shelled trilobites with large pygidia (only partially nektonic) |
||||||
Cyclopygidæ | ![]() |
(nektonic dysphotic) | ||||||
Remopleuridæ | ||||||||
Benthonic | ![]() |
Crawlers and slow swimmers | Most trilobites with small pygidia. Triarthrus, Paradoxides, etc. | |||||
Crawlers and slow swimmers | Most trilobites with large pygidia. Isotelus, Dalmanites, etc. | |||||||
Crawlers and slow swimmers | Trilnuceidæ, Harpedidæ, some Mesonacidæ, etc. |
This subject has been less discussed than the methods of locomotion. The study of the appendages has shown that while the mouth parts were not especially powerful, they were at least numerous, and sufficiently armed with spines to shred up such animal and vegetable substances as they were liable to encounter. It having been ascertained that the shape of the glabella and axial lobe furnishes an indication of the degree of development of the alimentary canal it is possible to infer something of the kind of food used by various trilobites.
The narrow glabellæ and axial lobes of the oldest trilobites would seem to indicate a carnivorous habit, while the swollen glabellæ and wider lobes of later ones probably denote an adaptation to a mixed or even a vegetable diet. This can not be relied upon too strictly, of course, for the swollen glabellæ of such genera as Deiphon or Sphærexochus may be due merely to the shortening up of the cephalon.
Walcott (1918, p. 125) suggests that the trilobites lived largely upon worms and conceives of them as working down into the mud and prowling around in it in search of such prey. While there can be no doubt that many trilobites had the power of burying themselves in loose sand or mud, a common habit with modern crustaceans, most of them were of a very awkward shape for habitual burrowers, and how an annelid could be successfully pursued through such a medium by an animal of this sort is difficult to understand. In fact, the presence of the large hypostoma and the position of the mouth were the great handicaps of the trilobite as a procurer of live animal food, and coupled with the relatively slow means of locomotion, almost compel the conclusion that errant animals of any size were fairly safe from it. This restricts the range of animal food to small inactive creatures and the remains of such larger forms as died from natural causes. The modern Crustacea are effective scavengers, and it is probable that their early Palæozoic ancestors were equally so. It is a common saying that in the present stressful stage of the world's history, very few wild animals die a natural death. In Cambrian times, competition for [104] animal food was less keen, and with the exception of a few cephalopods, a few large annelids, and a few Crustacea like Sidneyia, there seem to have been no aggressive carnivores. In consequence, millions of animals must have daily died a natural death, and had there been no way of disposing of their remains, the sea bottom would soon have become so foul that no life could have existed. For the work of removal of this decaying matter, the carnivorous annelids and the Crustacea, mostly trilobites, were the only organisms, and it is probable that the latter did their full share. After prowling about and locating a carcass, the trilobite established himself over it, the cephalon and hypostoma on one end and the pygidium on the other enclosing and protecting the prey, which was shredded off and passed to the mouth at leisure by means of the spinose endobases.
Even in Middle Cambrian times some trilobites (e. g., Paradoxides) seem to have enlarged the capacity of the stomach and taken vegetable matter, but later, in the Upper Cambrian and Ordovician, when the development of cephalopods and fishes caused great competition for all animal food, dead or alive, most trilobites seem to have become omnivorous. This is of course shown by the swollen glabella, with reduced lateral furrows, and, in the case of a few species (Calymene, Ceraurus), the known enlargement of the stomach.
Cryptolithus is the only trilobite which has furnished any actual evidence as to its food. From the fact that the alimentary tract is found stuffed from end to end with fine mud, and because it is known to have been a burrower, it has been suggested by several that it was a mud feeder, passing the mud through the digestive tract for the sake of what organic matter it contained. Spencer (1903, p. 491) has suggested a modification of this view:
The phyllopods appear to feed by turning over whilst swimming and seizing with their more posterior appendages a little mud which swarms with infusoria, etc. This mud is then pushed along the ventral groove to the mouth. Casts, of the intestine of trilobites are still found filled with the mud.
Ceraurus and Calymene also must have occasionally swallowed mud in quantity, otherwise the form of the alimentary canal could not have been preserved as it is in a few of Doctor Walcott's specimens.
TRACKS AND TRAILS OF TRILOBITES.
Tracks and trails of various sorts have been ascribed by authors to trilobites since these problematic markings first began to attract attention, but as the appendages were until recently quite unknown, all the earlier references were purely speculative. The subject is a difficult one, and proof that any particular track or trail could have been made in only one way is not easily obtained. Since the appendages have actually been described, comparatively little has been done, Walcott's work on Protichnites (1912 B, p. 275) being the most important. Since the first description of Protichnites by Owen (Quart. Jour. Geol. Soc., London, 1852, vol. 8, p. 247), it has been thought that these trails were made by crustaceans, and the only known contemporaneous crustaceans being trilobites, these animals were naturally suggested. Dawson (Canadian Nat. Geol., vol. 7, 1862, p. 276) ascribed them, with reserve, to Paradoxides, and Billings (1870, p. 484) suggested Dikelocephalus or Aglaspis. Walcott secured well preserved specimens which showed trifid tracks, and these were readily explained when he found the legs of Neolenus, which terminated with three large spines. Similar trifid terminations had already been described by Beecher, and clearly pictured in his restoration of Triarthrus, but the spines and the tracks had somehow not previously been connected in the mind of any observer. Walcott concluded that the tracks had been made [105] by a species of Dikelocephalus, possibly by D. hartti, which occurs both north and south of the Adirondacks. In a recent paper, Burling (Amer. Jour. Sci., ser. 4, vol. 44, 1917, p. 387) has argued that Protichnites was not the trail of a trilobite, but of a "short, low-lying, more or less heavy set, approximately 12-legged, crab-like animal," which had an oval shape, toed in, and was either extremely flexible or else short and more or less flexible in outline. This seems to describe a trilobite.
Climactichnites, the most discussed single trail of all, has also been ascribed to trilobites,—by Dana (Manual of Geology, 1863, p. 185), Billings (1870, p. 485), and Packard (Proc. Amer. Acad. Arts and Sci., vol. 36, 1900, p. 64),—though less frequently than to other animals. The latest opinion (see paper by Burling cited above) seems to be against this theory.
Miller (1880, p. 217) described under the generic name Asaphoidichnus two kinds of tracks which were such as he supposed might be made by an Asaphus (Isotelus). In referring to the second of the species, he says: "Some of the toe-tracks are more or less fringed, which I attribute to the action of water, though Mr. Dyer is impressed with the idea that it may indicate hairy or spinous feet." The type of this species, A. dyeri, is in the Museum of Comparative Zoology, and while it may be the trail of a trilobite, it would be difficult to explain how it was produced.
Ringueberg (1886, p. 228) has described very briefly tracks found in the upper part of the Medina at Lockport, New York. These consisted of a regularly succeeding series of ten paired divergent indentations arranged in two diverging rows, with the trail of the pygidium showing between each series. The ten pairs of indentations he considered could have been made by ten pairs of legs like those shown by the specimen of Isotelus described by Mickleborough, and the intermittent appearance of the impression of the pygidium suggested to him that the trilobite proceeded by a series of leaps.
Walcott (1918, pp. 174-175, pl. 37-42) has recently figured a number of interesting trails as those of trilobites, and has pointed out that a large field remains open to anyone who has the patience to develop this side of the subject.
PART III.
RELATIONSHIP OF THE TRILOBITES TO OTHER ARTHROPODA.
It can not be said that the new discoveries of appendagiferous trilobites have added greatly to previous knowledge of the systematic position of the group. Probably none will now deny that trilobites are Crustacea, and more primitive and generalized than any other group in that class. The chief interest at present lies in their relation to the most nearly allied groups, and to the crustacean ancestor.
Trilobites have been most often compared with Branchiopoda, Isopoda, and Merostomata, the present concensus of opinion inclining toward the notostracan branchiopods (Apodidæ in particular) as the most closely allied forms. It seems hardly worth while to burden these pages with a history of opinion on this subject, since it was not until the appendages were fully made out, from 1881 to 1895, that zoologists and palæontologists were in a position to give an intelligent judgment. The present status is due chiefly to Bernard (1894), Beecher (1897, 1900, et seq.), and Walcott (1912, et seq.).
The chief primitive characteristics of trilobites are: direct development from a protaspis common to the subclass; variability in the number of segments, position of the mouth, and type of eyes; and serially similar biramous appendages.
The recent study has modified the last statement slightly, since it appears that in some trilobites there was a modification of the appendages about the mouth, suggesting the initiation of a set of tagmata.
In comparing the trilobites with other Crustacea, the condition of the appendages must be especially borne in mind, for while these organs are those most intimately in contact with the environment, and most subject to modification and change, yet they have proved of greatest service in classification.
Appendages have been found on trilobites from only the Middle Cambrian and Middle and Upper Ordovician, but as the Ordovician was the time of maximum development of the group, it is probable that trilobites of later ages would show degradational rather than progressive changes. All the genera which are known show appendages of the same plan, and although new discoveries will doubtless reveal many modifications of that plan, general inferences may be drawn now with some assurance.
The chief characteristics of the appendages are: first, simple antennules, a primitive feature in all Crustacea, as shown by ontogeny; second, paired biramous appendages, similar to each other all along the body, the youngest and simplest in front of the anal segment, the oldest and most modified on the cephalon. The endobases are retained on all the coxopodites, except possibly, in some species, the anterior ones, and these gnathobases are modified in some genera as mouth-parts, while in others they are similar throughout the series. With these few fundamentals in mind, other Crustacea may be examined for likenesses. The differences are obvious.
Crustacea.
The early idea that the trilobites were closely related to the Branchiopoda was rejuvenated by the work of Bernard on the Apodidæ (1892) and has since received the support [107] of most writers on the subject. Fundamentally, a great deal of the argument seems to be that Apus lies the nearest of any modern representative of the class to the theoretical crustacean ancestor, and as the trilobites are the oldest Crustacea, they must be closely related. Most writers state that the trilobites could not be derived from the Branchiopoda (see, however, Walcott 1912 A), nor the latter from any known trilobite, but both subclasses are believed to be close to the parent stem.
Viewed from the dorsal side, there is very little similarity between any of the branchiopods and the trilobites, and it is only in the Notostraca, with their sessile eyes and depressed form, that any comparison can be made. The chief way in which modern Branchiopoda and Trilobita agree is that both have a variable number of segments in the body, that number becoming very large in Apus on the one hand and Mesonacis and Pædeumias on the other. In neither are the appendages, except those about the mouth, grouped in tagmata. Other likenesses are: the Branchiopoda are the only Crustacea, other than Trilobita, in which gnathobases are found on limbs far removed from the mouth; the trunk limbs are essentially leaf-like in both, though the limb of the branchiopod is not so primitive as that of the trilobite; caudal cerci occur in both groups.
If the appendages be compared in a little more detail, the differences prove more striking than the likenesses.
In the Branchiopoda, the antennules are either not segmented or only obscurely so. In trilobites they are richly segmented.
In Branchiopoda, the antennæ are variable. In the Notostraca they are vestigial, while in the males of the Anostraca they are powerful and often complexly developed claspers. Either condition might develop from the generalized biramous antennas of Trilobita, but the present evidence indicates a tendency toward obsolescence. Claus' observations indicate that the antennæ of the Anostraca are developments of the exopodites, rather than of the endopodites.
The mandibles and maxillæ of the Branchiopoda are greatly reduced, and grouped closely about the mouth. Only the coxopodites of the Trilobita are modified as oral appendages.
The trunk limbs of Apus are supposed to be the most primitive among the Branchiopoda, and comparison will be made with them. Each appendage consists of a flattened axial portion, from the inner margin of which spring six endites, and from the outer, two large flat exites (see fig. 34). This limb is not articulated with the ventral membrane, but attached to it, and, if Lankester's interpretation of the origin of schizopodal limbs be correct, then the limb of Apus bears very little relation to that of the Trilobita. In Apus there is no distinct coxopodite and the endobases which so greatly resemble the similar organs in the Trilobita are not really homologous with them, but are developments of the first endite. Beecher's comparison of the posterior thoracic and pygidial limbs of Triarthrus with those of Apus can not be sustained. Neither Triarthrus nor any other trilobite shows any trace of phyllopodan limbs. Beecher figured (1894 B, pl. 7, figs. 3, 4) a series of endopodites from the pygidium of a young Triarthrus beside a series of limbs from a larval Apus. Superficially, they are strikingly alike, but while the endopodites of Triarthrus are segmented, the limbs of Apus are not, and the parts which appear to be similar are really not homologous. The similarity of the thoracic limbs in the two groups is therefore a case of parallelism and does not denote relationship.
Geologically, the Branchiopoda are as old as the Trilobita, and while they did not have the development in the past that the trilobite had, they were apparently differentiated fully as early. Anostraca, Notostraca and Conchostraca, three of the four orders, are represented in the Cambrian by forms which are, except in their appendages, as highly organized as the existing species. Brief notes on the principal Middle Cambrian Branchiopoda follow:
Illustrated: Walcott, Smithson. Misc. Coll., vol. 57, 1912, p. 177, pl. 27, figs. 1-3; pl. 30, figs. 3, 4.
This is the most strikingly like the modern Branchiopoda of any species described by Walcott from the Middle Cambrian, and invites comparison with Apus. The carapace is long, loosely attached to the body, and extends over the greater part of the thorax. The eyes are small, sessile, and close to the anterior margin.
The appendages of the head consist of two pairs of antennæ, and three pairs of slender, jointed legs. Both pairs of antennæ are slender and many-jointed, the antennules somewhat smaller than the antennæ. The exact structure of the limbs about the mouth has not yet been made out, but they are slender, tapering, endopodite-like legs, with at least three or four segments in each, and probably more.
There are eight pairs of thoracic appendages, each limb having the form of the endopodite of a trilobite and consisting of seven segments and a terminal spine. The proximal three segments of each appendage are larger than the outer ones, and have a flattened triangular expansion on the inner side. Walcott also states that "One specimen shows on seven pairs of legs, small, elongate, oval bodies attached near the first joint to the outer side of the leg. These bodies left but slight impression on the rock and are rarely seen. They appear to represent the gills." They are not figured, but taken in connection with the endopodite-like appearance of the segmented limbs, one would expect them to be vestigial exopodites.
A small hypostoma is present on the ventral side, and several of the specimens show wonderfully well the form of the alimentary canal and the hepatic cæca. The main branches of the latter enter the mesenteron just behind the fifth pair of cephalic appendages.
Behind the thorax the abdomen is long, limbless, and tapers to a point. It is said to consist of at least thirty segments.
Compared with Apus, Burgessia appears both more primitive and more specialized. The carapace and limbless abdomen are Apus-like, but there are very few appendagiferous segments, and the appendages are not at all phyllopodan, but directly comparable with those of trilobites, except, of course, for the uniramous character of the cephalic limbs. A closer comparison may be made with Marrella.
Illustrated: Walcott, Smithson. Misc. Coll., vol. 57, 1912, p. 181, pl. 27, figs. 4, 5.
The carapace is short, covering the head and the anterior part of the thorax. The latter consists of eight short segments with appendages, while the six abdominal segments, which are similar to those of the thorax, are without limbs except for the last, which bears a pair of broad swimmerets. The eyes are marginal and pedunculate. The antennules are imperfectly known, but apparently short, while the antennas are long and slender, with relatively few, long, segments. The mandibles appear to be like endopodites of trilobites and show at least six segments. As so often happens in these specimens from British Columbia, [109] the preservation of the other appendages is unsatisfactory. As illustrated (Walcott, 1912 A, pl. 27, fig. 5), both endopodites and exopodites appear to be present, and the shaft of the exopodite seems to be segmented as in Triarthrus.
Walcott considers Waptia as a transitional form between the Branchiopoda and the Malacostraca.
Illustrated: Walcott, Smithson. Misc. Coll., vol. 57, 1912, p. 172, pl. 29, figs. 7-13.
This species, though incompletely known, has several interesting characteristics. The head shows, quite plainly in some specimens, the five segments of which it is composed. The eyes are small, situated in a niche between the first and second segments, and are described as being pedunculate. The eight segments of the thorax all show short triangular pleural extensions, somewhat like those of Remopleurides or Robergia. The abdomen consists of four cylindrical segments, the last with a pair of expanded caudal rami.
The antennules appear to be short, while the antennas are large, with several segments, ending in three spines, and apparently adapted for serving as claspers in the male. The third, fourth, and fifth pairs of cephalic appendages are short, tapering, endopodite-like legs similar to those of Burgessia.
The appendages of the thorax are not well preserved, and there seem to be none on the abdomen.
This species is referred by Walcott to the Anostraca.
Illustrated: Walcott, Smithson. Misc. Coll., vol. 57, 1912, p. 167, pl. 27, fig. 6; pl. 28, fig. 1.
This most remarkably specialized anostracan is not well enough known to allow comparison to be made with other contemporaneous Crustacea, but it is worthy of mention.
There is no carapace, the eyes are pedunculated, thorax and abdomen are not differentiated, and the telson is a broad, elongate, spatulate plate. There seem to be sexual differences in the form of the anterior cephalic and caudal appendages, but this is not fully established. The most remarkable feature is the long, large, median cephalic appendage which is so suggestive of the proboscis of the recent Thamnocephalus platyurus Packard. The appendages are not well enough preserved to permit a determination as to whether they are schizopodal or phyllopodan.
Walcott referred Burgessia and Waptia to new families under the Notostraca, while Yohoia and Opabina were placed with the Anostraca. Except for the development of the carapace, there is a striking similarity between Waptia and Yohoia, serving to connect the two groups.
The Branchiopoda were very highly specialized as early as Middle Cambrian time, the carapace of the Notostraca being fully developed and the abdomen limbless. Some (Burgessia) had numerous segments, but most had relatively few. The most striking point about them, however, is that so far as is known none of them had phyllopodan limbs. While the preservation is in most cases unsatisfactory, such limbs as are preserved are trilobite-like, and in the case of Burgessia there can be no possible doubt of the structure. Another interesting feature is the retention by Yohoia of vestiges of pleural lobes. The Middle [110] Cambrian Branchiopoda are more closely allied to the Trilobita than are the modern ones, but still the subclass is not so closely related to that group as has been thought. Modern Apus is certainly much less like a trilobite than has been supposed, and very far from being primitive. The Branchiopoda of the Middle Cambrian could have been derived from the trilobites by the loss of the pleural lobes, the development of the posterior margin of the cephalon to form a carapace, and the loss of the appendages from the abdominal segments. Modern branchiopods can be derived from those of the Middle Cambrian by the modification of the appendages through the reduction of the endopodite and exopodite and the growth of the endites and exites from the proximal segments.
Carpenter (1903, p. 334), from his study of recent crustaceans, has already come to the conclusion that the Branchiopoda are not the most primitive subclass, and this opinion is strengthened by evidence derived from the Trilobita and from the Branchiopoda of the Middle Cambrian.
The non-parasitic Eucopepoda are in many ways much nearer to the trilobites than any other Crustacea. These little animals lack the carapace, and the body is short, with typically ten free segments and a telson bearing caudal furcæ. The head is composed of five segments (if the first thoracic segment is really the fused first and second), is often flattened, and lacks compound eyes. Pleural lobes are well developed, but instead of being flattened as in the trilobite, they are turned down at the sides or even incurved. A labrum is present.
The antennules, antennæ, and mandibles are quite like those of trilobites. The antennules are very long and made up of numerous segments. The antennæ are biramous, the junction between the coxopodite and basipodite is well marked, and the endopodite consists of only two segments.
The mandibles are said to "retain more completely than in any other Crustacea the form of biramous swimming limbs which they possess in the nauplius." The coxopodites form jaws, while both the reduced endopodite and exopodite are furnished with long setæ. The maxillulæ are also biramous, but very different in form from those of the trilobite, and the maxillæ are phyllopodan.
The first thoracic limb is uniramous and similar to the maxillæ, but the five following pairs are biramous swimming legs with coxopodite, basipodite, exopodite, and endopodite. Both the exopodite and endopodite are shorter than in the trilobites, but bear setæ and spines.
The last pair of thoracic limbs are usually modified in the male into copulatory organs. In some females they are enlarged to form plates for the protection of the eggs, in others they are unmodified. In still others they are much reduced or disappear. The abdomen is without appendages.
The development in Copepoda is direct, by the addition posteriorly to the larval form (nauplius) of segments, and the appendages remain nearly unmodified in the adult.
Altogether, the primitive Copepoda seem much more closely allied to the Trilobita than any other modern Crustacea, but unfortunately no fossil representative of the subclass has been found. This is not so surprising when one considers the habits and the habitat of most of the existing species. Many are parasitic, many pelagic in both fresh and marine waters, and many of those living on the bottom belong to the deep sea or fresh water. Most free-living forms are minute, and all have thin tests.
The eyes of copepods are of interest, in that they suggest the paired ocelli of the Harpedidæ and Trinucleidæ. In the Copepoda there are, in the simplest and typical form of these organs, three ocelli, each supplied with its own nerve from the brain. Two of these are dorsal and look upward, while the third is ventral. In some forms the dorsal ocelli are doubled, so that five in all are present (cf. some species of Harpes with three ocelli on each mound). In some, the cuticle over the dorsal eyes is thickened so as to form a lens, as appears to be the case in the trilobites. These peculiar eyes may be a direct inheritance from the Hypoparia.
Professor Schuchert has called my attention to the exceedingly curious little crustacean which Handlirsch (1914) has described from the Triassic of the Vosges. Handlirsch erected a new species, genus, family, and order for this animal, which he considered most closely allied to the copepods, hence the ordinal name. Euthycarcinus kessleri, the species in question, was found in a clayey lens in the Voltzia sandstone (Upper Bunter). Associated with the new crustacean were specimens of Estheria only, but in the Voltzia sandstone itself land plants, fresh and brackish water animals, and occasionally, marine animals are found. The clayey lens seems to have been of fresh or brackish water origin.
All of the specimens (three were found) are small, about 35 mm. long without including the caudal rami, crushed flat, and not very well preserved. The head is short, not so wide as the succeeding segments, and apparently has large compound eyes at the posterior lateral angles. The thorax consists of six segments which are broader than the head or abdomen. The abdomen, which is not quite complete in any one specimen, is interpreted by Handlirsch as having four segments in the female and five in the male. Least satisfactory of all are traces of what are interpreted by the describer as a pair of long stiff unsegmented cerci or stylets on the last segment.
The ventral side of one head shield shows faint traces of several appendages which must have presented great difficulty in their interpretation. A pair of antennules appear to spring from near the front of the lower surface, and the remainder of the organs are grouped about the mouth, which is on the median line back of the center. Handlirsch sees in these somewhat obscure appendages four pairs of biramous limbs, antennæ, mandibles, maxillulæ, and maxillæ, both branches of each consisting of short similar segments, endopodites and exopodites being alike pediform.
Each segment of the thorax has a pair of appendages, and those on the first two are clearly biramous. The endopodites are walking legs made up of numerous short segments (twelve or thirteen according to Handlirsch's drawing), while the exopodite is a long breathing and rowing limb, evidently of great flexibility and curiously like the antennules of the same animal. The individual segments are narrow at the proximal end, expand greatly at the sides, and have a concave distal profile. A limb reminds one of a stipe of Diplograptus. Both branches are spiniferous.
No appendages are actually present on the abdomen, but each segment has a pair of scars showing the points of attachment. From the small size of these, it is inferred that the limbs were poorly developed.
This species is described in so much detail because, if it is a primitive copepod, it has a very important bearing on the ancestry of that group and is the only related form that has been found fossil.
The non-parasitic copepods have typically ten (eleven) free segments, including the telson, and the four abdominal segments are much more slender than the six in front of them. In this respect the agreement is striking, and the presence of five pairs of appendages in the head and six free segments in the thorax is a more primitive condition than in modern forms where the first two thoracic segments are apparently fused (Calman, 1909, p. 73).
The large compound eyes of this animal are of course not present in the copepods, but as vestiges of eyes have been found in the young of Calanus, it is possible that the ancestral forms had eyes.
The greatest difficulty is in finding a satisfactory explanation of the appendages. The general condition is somewhat more primitive than in the copepods, for all the appendages are biramous, while in the modern forms the maxillipeds are uniramous and the sixth pair of thoracic appendages are usually modified in the male as copulatory organs. In the copepods the modification is in the direction of reduction, both endopodites and exopodites usually possessing fewer segments than the corresponding branches in the trilobites. The endopodite of Euthycarcinus, on the contrary, possesses, if Handlirsch's interpretation is correct, twice as many segments as the endopodite of a trilobite. If the Copepoda are descended from the trilobites, as everything tends to indicate, then Euthycarcinus is certainly not a connecting link. The only truly copepodan characteristic of this genus is the agreement in number and disposition of free segments. The division into three regions instead of two, the compound eyes, and the structure of the appendages are all foreign to that group.
With the Limulava fresh in mind, one is tempted to compare Euthycarcinus with that ancient type. The short head and large marginal eyes recall Sidneyia, and the grouping of the appendages about the mouth also suggests that genus and Emeraldella. In the Limulava likewise there is a contraction of the posterior segments, although it is behind the ninth instead of the sixth. There is no likeness in detail between the appendages of the Limulava and those of Euthycarcinus, but the composite claws of Sidneyia show that in this group there was a tendency toward the formation of extra segments.
If this fossil had been found in the Cambrian instead of the Triassic, it would probably have been referred to the Limulava, and is not at all impossible that it is a descendant from that group. As a connecting link between the Trilobita and Copepoda it is, however, quite unsatisfactory.
The bivalved shell of the Ostracoda gives to this group of animals an external appearance very different from that of the trilobites, but the few appendages, though highly modified, are directly comparable. The development, although modified by the early appearance of the bivalved shell within which the nauplius lies, is direct. Imperfect compound eyes are present in one family.
The antennules are short and much modified by functioning as swimming, creeping, or digging organs. They consist of eight or less segments. The antennas are also locomotor organs, and in most orders are biramous. The mandibles are biramous and usually with, but sometimes without, a gnathobase. The maxillulæ are likewise biramous but much modified.
The homology of the third post-oral limb is in question, some considering it a maxilla and others a maxilliped. It has various forms in different genera. It is always much modified, [113] but exopodite and endopodite are generally represented at least by rudiments. The fourth post-oral limb is a lobed plate, usually not distinctly segmented, and the fifth a uniramous pediform leg. The sixth, if present at all, is vestigial.
Very little comparison can be made between the Ostracoda and Trilobita, other than in the ground-plan of the limbs, but the presence of biramous antennæ is a primitive characteristic.
Like the ostracod, the adult cirriped bears little external resemblance to the trilobite. The form of the nauplius is somewhat peculiar, but it has the typical three pairs of appendages, to which are added in the later metanauplius stages the maxillæ and six pairs of thoracic appendages. In the adult, the antennules, which serve for attachment of the larva, usually persist in a functionless condition, while the antennas disappear. The mandibles, maxillulæ, and maxillæ are simple and much modified to form mouth parts, and the six pairs of thoracic appendages are developed into long, multisegmented, biramous appendages bearing numerous setæ which serve for catching prey. Paired eyes are present in later metanauplius stages, but lost early in the development. The relationship to the trilobite evidently is not close.
The oldest malacostracans whose appendages are known are species of Hymenocaris. One, described as long ago as 1866 by Salter, has what seem to be a pair of antennæ and a pair of jaw-like mouth-parts. Another more completely known species has recently been reported by Walcott (1912 A, p. 183, pl. 31, figs. 1-6). This latter form is described as having five pairs of cephalic appendages: a pair of minute antennules beside the small pedunculated eyes, a pair of large uniramous antennæ, slender mandibles and maxillulæ, and large maxillæ composed of short stout segments. There are eight pairs of biramous thoracic limbs, the exopodites setiferous, the endopodites composed of short wide segments and ending in terminal claw-like spines. These appendages are like those of trilobites.
Hymcnocaris belongs to the great group of extinct ceratocarid Crustacea which are admitted to the lowest of the malacostracan orders, Phyllocarida, because of their resemblance to Nebalia, Paranebalia, Nebaliopsis, and Nebaliella, the four genera which are at present living. The general form of the recent and fossil representatives of the order is strikingly similar. The chief outward difference is that in many of the fossils the telson is accompanied by two furcal rami, while in the modern genera it is simple. It now becomes possible to make some comparison between the appendages of Hymcnocaris of the Middle Cambrian and the Nebaliidæ of modern seas.
In both there are five pairs of cephalic and eight of thoracic appendages, while those of the abdomen of Hymenocaris are not known.
In both, the antennules are less developed than the antennæ. In the Nebaliidæ the antennules show evidence of having been originally double (they are obviously so in the embryo), while they are single in Hymcnocaris. In both, the antennæ are simple. The remaining cephalic organs are too little shown by the specimen from the Middle Cambrian to allow detailed comparison. The mandibles, maxillulæ, and maxillæ of Nebalia are, however, of types which could be derived from the trilobite.
In three of the genera of the Nebaliidæ, the eight pairs of thoracic limbs are all similar to one another, though those of the genera differ. All are biramous. The limbs of Hymcnocaris can apparently be most closely correlated with those of Nebalia antarctica, in which the endopodite consists of short flattened segments, and the exopodite is a long setiferous plate. Epipodites are present in both Nebalia and Hymcnocaris.
So far as the appendages of Hymenocaris are known, they agree very well with those of the Nebaliidæ, and since they are of the trilobite type, it may safely be stated that the Trilobita and Malacostraca are closely related.
Walcott (1918, p. 170) has compared the limbs of Neolenus with those of the syncarid genera Anaspides and Koonunga. These are primitive Malacostraca without a carapace, but as they have a compressed test and Anaspides has stalked eyes, their gross anatomy does not suggest the trilobite. The thoracic appendages are very trilobite-like, since the endopodite has six segments (in Anaspides) and a multisegmented setiferous exopodite. The coxopodites, except of the first thoracic segment, do not, however, show endobases, and those which are present are peculiar articulated ones. The cephalic appendages are specialized, and the antennules double as in most of the Malacostraca. External epipodites are very numerous on the anterior limbs.
This group extends back as far as the Pennsylvanian and had then probably already become adapted to fresh-water life. It may be significant that the Palæozoic syncarids appear to have lacked epipodites. While differing very considerably from the Trilobita, the Syncarida could have been derived from them.
Since the earliest times there has been a constant temptation to compare the depressed shields of the trilobites with the similar ones of isopods. Indeed, when Scrolls with its Lichadian body was first discovered about a hundred years ago, it was thought that living trilobites had been found at last. The trilobate body, cephalic shield, sessile eyes, abdominal shield, and pleural extensions make a wonderful parallel. This similarity is, however, somewhat superficial. The appendages are very definitely segregated in groups on the various regions of the body, and while the pleopods are biramous, the thoracic legs are without exopodites (except in very early stages of development of one genus). The Isopoda arose just at the time of the disappearance of the Trilobita, and there seems a possibility of a direct derivation of the one group from the other. It should be pointed out that while the differences of Isopoda from Trilobita are important, they are all of a kind which could have been produced by the development from a trilobite-like stock. For example:
Isopoda have a definite number of segments. There is less variation in the number of segments among the later than the earlier trilobites.
Isopoda have no facial suture. In at least three genera of trilobites the cheeks become fused to the cranidium and the sutures obliterated.
Isopoda have one or two segments of the thorax annexed to the head. While this is not known to occur in trilobites, it is possible that it did.
Most Isopoda have a fairly stiff ventral test. The ventral membrane of trilobites would probably have become stiffened by impregnation of lime if the habit of enrollment had been given up.
In Isopoda the antennæ are practically uniramous sensory organs. The second cephalic appendages of trilobites are capable of such development through reduction of the exopodite.
In the Isopoda the coxopodites are usually fused with the body, remaining as free, movably articulated segments only in a part of the thoracic legs of one suborder, the Asellota. Endobases are entirely absent. This is of course entirely unlike the condition in Trilobita, but a probable modification.
In Isopoda there is a distinct grouping of the appendages, with specialization of function. The trilobites show a beginning of tagmata, and such development would be expected if evolution were progressive.
In both groups, development from the embryo is direct. Rudiments of exopodites of thoracic legs have been seen in the young of one genus.
The oldest known isopod is Oxyuropoda ligioides Carpenter and Swain (Proc. Royal Irish Acad., vol. 27, sect. B, 1908, p. 63, fig. 1), found in the Upper Devonian of County Kilkenny, Ireland. The appendages are not known, but the test is in some ways like that of a trilobite. The thorax, abdomen, and pygidium are especially like those of certain trilobites, and there is no greater differentiation between thorax and abdomen than there is between the regions before and behind the fifteenth segment of a Pædeumias or Mesonacis. The anal segment is directly comparable to the pygidium of a Ceraurus, the stiff unsegmented uropods being like the great lateral spines of that genus.
The interpretation of the head offered by Carpenter and Swain is very difficult to understand, as their description and figure do not seem to agree. What they consider the first thoracic segment (fused with the head) seems to me to be the posterior part of the cephalon. and it shows at the back a narrow transverse area which is at least analogous to the nuchal segment of the trilobite. If this interpretation can be sustained, Oxyuropoda would be a very primitive isopod in which the first thoracic segment (second of Carpenter and Swain) is still free. According to the interpretation of the original authors, the species is more specialized than recent Isopoda, as they claim that two thoracic segments are fused in the head. The second interpretation was perhaps made on the basis of the number of segments (nineteen) in a recent isopod.
Illustrated: Walcott, Smithson. Misc. Coll., vol. 57, 1912, p. 192, pls. 25, 26.
Among the most wonderful of the specimens described by Doctor Walcott is the "lace crab." While the systematic position was not satisfactorily determined by the describer, it has been aptly compared to a trilobite. The great nuchal and genal spines and the large marginal sessile eyes, coupled with the almost total lack of thoracic and abdominal test, give it a bizarre appearance which may obscure its real relationships.
The cephalon appears to bear five pairs of appendages, antennules, and antennæ, both tactile organs with numerous short segments, mandibles, and first and second maxillæ. The last three pairs are elongate, very spinose limbs, of peculiar appearance. They seem to have seven segments, but are not well preserved. These organs are attached near the posterior end of the labrum.
There are twenty-four pairs of biramous thoracic appendages, which lack endobases. The endopodites are long and slender, with numerous spines; the exopodites have narrow, thin shafts, with long, forward pointed setæ. The anal segment consists of a single plate.
Further information about this fossil will be eagerly awaited. None of the illustrations so far published shows biramous appendages on the cephalon. This, coupled with the presence of tactile antennæ, makes its reference to the Trilobita impossible, but the present interpretation indicates that it was closely allied to them.
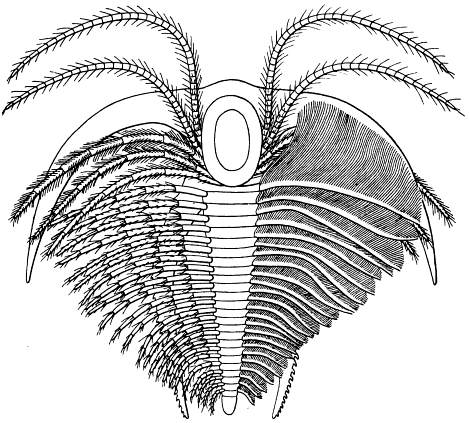
Fig. 32. Marrella splendens Walcott. Restoration of the ventral surface, based upon the photographs and descriptions published by Walcott. Although all the limbs of the trunk appear to be biramous, only endopodites are placed on one side and exopodites on the other, for the sake of greater clearness in the illustration. Drawn by Doctor Elvira Wood, under the supervision of the writer. × about 6.
(Text fig. 32.)
The accompanying restoration of the ventral surface of Marrella is a tentative one, based on Doctor Walcott's description and figures. The outline is taken from his plate 26, figure 1; the appendages of the head from plate 26, figures 1-3, 5, and plate 25, figures 2, 3; the endopodites, shown on the left side only, from figures 3 and 6, plate 25. I have not studied actual specimens, and the original description is very incomplete. The restoration is therefore subject to revision as the species becomes better known.
Arachnida.
No attempt will be made to pass in review all of the subclasses of the arachnids. Some of the Merostomata are so obviously trilobite-like that it would seem that their relationship could easily be proved. The task has not yet been satisfactorily accomplished, however, and new information seems only to add to the difficulties.
So far as I know, the Araneæ have not previously been compared directly with trilobites, although such treatment consists merely in calling attention to their crustacean affinities, as has often been done.
Carpenter's excellent summary (1903, p. 347) of the relationship of the Arachnida to the trilobites may well be quoted at this point:
The discussion in a former section of this essay on the relationship between the various orders of Arachnida led to the conclusion that the primitive arachnids were aquatic animals, breathing by means of appendicular gills. Naturally, therefore, we compare the arachnids with the Crustacea rather than with the Insecta. The immediate progenitors of the Arachnida appear to have possessed a head with four pairs of limbs, a thorax with three segments, and an abdomen with thirteen segments and' a telson, only six of which can be clearly shown by comparative morphology to have carried appendicular gills. But embryological evidence enables us to postulate with confidence still more remote ancestors in which the head carried well developed compound eyes and five pairs of appendages, while it may be supposed that all the abdominal segments, except the anal, bore limbs. In these very ancient arthropods, all the limbs, except the feelers, had ambulatory and branchial branches; and one important feature in the evolution of the Arachnida must have been the division of labour between the anterior and posterior limbs, the former becoming specialized for locomotion, the latter for breathing. Another was the loss of feelers and the degeneration of the compound eyes. Thus we are led to trace the Arachnida (including the Merostomata and Xiphosura) back to ancestors which can not be regarded as arachnids, but which were identical with the primitive trilobites, and near the ancestral stock of the whole crustacean class.
While no one having any real knowledge of the Trilobita has adopted Lankester's scheme of the inclusion of the group as the primitive grade in the Arachnida, reference to it may not be amiss. This theory is best set forth in the Encyclopædia Britannica, Eleventh Edition, under the article on Arachnida. It is there pointed out that the primitive arachnid, like the primitive crustacean, should be an animal without a fixed number of somites, and without definitely grouped tagmata. As Lankester words it, they should be anomomeristic and anomotagmatic. The trilobites are such animals, and he considers them Arachnida and not Crustacea for the following reasons:
Firstly and chiefly, because they have only one pair (apart from the eyes) of pre-oral appendages. "This fact renders their association with the Crustacea impossible, if classification is to be the expression of genetic affinity inferred from structural coincidence."
Secondly, the lateral eyes resemble no known eyes so closely as the lateral eyes of Limulus.
Thirdly, the trilobation of the head and body, due to the expansion and flattening of the sides or pleura, is like that of Limulus, but "no crustacean exhibits this trilobite form."
Fourthly, there is a tendency to form a pygidial or telsonic shield, "a fusion of the posterior somites of the body, which is precisely identical in character with the metasomatic carapace of Limulus." No crustacean shows metasomatic fusion of segments.
Fifthly, a large post-anal spine is developed "in some trilobites" (he refers to a figure of Dalmanites).
Sixthly, there are frequently lateral spines on the pleura as in Limulus. No crustacean has lateral pleural spines.
These points may be taken up in order.
1. If trilobites have one appendage-bearing segment in front of the mouth, they are Arachnida; if two, Crustacea. This is based on the idea that in the course of evolution of the Arthropoda, the mouth has shifted backward from a terminal position, and that as a pair of appendages is passed, they lose their function as mouth-parts and eventually become simple tactile organs. Thus arise the cheliceræ of most arachnids, and the two pairs of tactile antennæ of most Crustacea. This theory is excellent, and the rule holds well for modern forms, but as shown by the varying length of the hypostoma in different trilobites, the position of the mouth had not become fixed in that group. In some trilobites, like Triarthrus, the gnathobases of the second pair of appendages still function, but in all, so far as known, the mouth was back of the points of attachment of at least two pairs of appendages, and in some at least, back of the points of attachment of four pairs. As pointed out in the case of Calymene and Ceraurus, the trilobites show a tendency toward the degeneration of the first and second pairs of biramous appendages, particularly of the gnathobases. They are in just that stage of the backward movement of the mouth when the function of the antennæ as mandibles has not yet been lost. If the presence of functional gnathobases back of the mouth, rather than the points of attachment in front of the mouth, is to be the guide, then Triarthrus might be classed as an arachnid and Calymene and Isotelus as crustaceans. In other words, the rule breaks down in this primitive group.
2. Superficially, the eyes of some trilobites do look like those of Limulus, but how close the similarity really was it is impossible to say. The schizochroal eyes were certainly very different, and Watase and Exner both found the structure of the eye of the trilobite unlike that of Limulus.
3. The importance of the trilobate form of the trilobite is very much overestimated. It and the pygidium are due solely to functional requirements. The axial lobe contained practically all the vital organs and the side lobes were mechanical in origin and secondarily protective. That the crustacean is not trilobate is frequently asserted by zoologists, yet every text-book contains a picture of a segment of a lobster with its axial and pleural lobes. It is a fundamental structure among the Crustacea, obscured because most of them are compressed rather than depressed.
4. The pygidium of trilobites is compared with the metasomatic shield of Limulus. No homology, if homology is intended, could be more erroneous. The metasomatic shield of Limulus is, as shown by ontogeny and phylogeny, formed by the fusion of segments formerly free, and includes the segments between the cephalic and anal shields, or what would be known as the thorax of a trilobite. No trilobite has a metasomatic shield. The pygidium of a trilobite, as shown by ontogeny, is built up by growth in front of the anal region, and since the segments were never free, it can not strictly be said to be composed of fused segments. Some Crustacea do form a pygidial shield, as in certain orders of the Isopoda.
5. The post-anal spine of Dalmanites and some other trilobites is similar to that of Limulus, but this seems a point of no especial significance. That a similar spine has not been developed in the Crustacea is probably due to the fact that they do not have the broad depressed shape which makes it so difficult for a Limulus to right itself when once turned on its back. Relatively few trilobites have it, and it is probably correlated with some special adaptation.
6. There is nothing among the trilobites comparable to the movable lateral spines of the metasoma of Limulus.
While, as classifications are made up, the Trilobita must be placed in the Crustacea rather than the Arachnida, there is no reason why both the modern Crustacea and the Arachnida should not be derived from the trilobites.
It has been a custom of long standing to compare the trilobite with Limulus. Packard (1872) gave great vitality to the theory of the close affinity of the two when he described the so called trilobite-stage in the development of Limulus polyphemus. His influence on Walcott's ideas (1881) is obvious. Lankester has gone still further, and associated the Trilobita with the Merostomata in the Arachnida.
The absence of antennules at any stage in development allies Limulus so closely with the Arachnida and separates it so far from the Trilobita that in recent years there has been a tendency to give up the attempt to prove a relationship between the merostomes and trilobites, especially since Clarke and Ruedemann, in their extensive study of the Eurypterida, found nothing to indicate the crustacean nature of that group. A new point of view is, however, presented by the curious Sidneyia inexpectans and Emeraldella brocki described by Walcott from the Middle Cambrian.
Illustrated: Walcott, Smithson. Misc. Coll., vol. 57, 1911, p. 21, pl. 2, fig. 1 (not figs. 2, 3); pls. 3-5; pl. 6, fig. 3; pl. 7, fig. 1.
The body of this animal is elongate, somewhat eurypterid-like, but with a broad telson supplied with lateral swimmerets. The cephalon is short, with lateral compound eyes. The trunk consists of eleven segments, the anterior nine of which are conspicuously wider than the two behind them, and the telson consists of a single elongate plate.
On the ventral side of the head there is a large hypostoma and five, pairs of appendages. The first pair are multisegmented antennules. The second pair have not been adequately described. The third are large, complex claws, and the fourth and fifth suggest broad, stocky endopodites. Broad gnathobases are attached to the coxopodites of the third to fifth pairs of appendages and form very strong jaws.
The first nine segments of the thorax have one pair each of broad filiform branchial appendages, suggestive of the exopodites of trilobites, but no endopodites have been seen. The tenth and eleventh segments seem to lack appendages entirely.
Illustrated: Sidneyia inexpectans Walcott partim, Smithson. Misc. Coll., vol. 57, 1911, pl. 2, figs. 2, 3 (not fig. 1);—Ibid., 1912, p. 206, text fig. 10.
Emeraldella brocki Walcott, Ibid., 1912, p. 203, pl. 30, fig. 2; text fig. 8;—Ibid., vol. 67, 1918, p. 118 (correction).
Emeraldella has much the same shape as Sidneyia and the same number of segments, but instead of a broad flat telson, it has a long Limulus-like spine. The cephalon is about as wide as long, and eyes have not yet been seen. The body consists of eleven segments and a telson (Walcott says twelve and a telson but shows only eleven in the figures). Nine of the segments, as in Sidneyia, are broad, the next two narrow.
The ventral side of the cephalon has a long hypostoma, and five pairs of appendages. The first pair are very long multi segmented antennules and the next four pairs seem to be rather slender, spiniferous, jointed endopodites. Whether or not gnathobases were present is not shown by the figures, but owing to the long hypostoma the appendages are grouped about the mouth. All the segments of the body, unless it were the telson, seem to have borne appendages. On the anterior end, they were clearly biramous (1912, p. 206, text fig. 10), and that they were present along the body is shown by figure 2, plate 30, 1912.
The present state of knowledge of both these peculiar animals leaves much to be desired. The indications are that the cephalic appendages are not biramous, and that only one pair of antennæ, the first, are developed as tactile organs. The thoracic appendages of Emeraldella are biramous, and also possibly those of Sidneyia. In the latter, the last two abdominal segments seem to have been without appendages, while in Emeraldella at least one branch of each appendage, and possibly both, is retained.
These animals, which may be looked upon as the last survivors of an order of pre-Cambrian arthropods, have the appearance of an eurypterid, but their dominant characteristics are crustacean. The features which suggest the Eurypterida are: elongate, obovate, non-trilobate, tapering body; telson-like posterior segment; marginal, compound, sessile eyes; claw-like third cephalic appendages; and, more particularly, the general resemblance of the test to that of an eurypterid like Strabops. In form, Sidneyia agrees with the theoretical prototype of the Eurypterida reconstructed by Clarke and Ruedemann (Mem. 14, N. Y. State Mus., vol. 1, 1912, p. 124) in its short wide head with marginal eyes, and its undifferentiated body. There is, moreover, no differentiation of the postcephalic appendages.
The crustacean characteristics are seen in the presence of five, instead of six, pairs of appendages on the head, the first of which are multisegmented antennules, and in the biramous appendages on the body of Emeraldella. It should be noted that these latter are typically trilobitic, each consisting of an endopodite with six segments and a setiferous exopodite.
Clarke and Ruedemann (1912, p. 406) have discussed Sidneyia briefly, and conclude:
It seems to us probable that the Limulava [Sidneyia and Amiella] as described are not eurypterids but constitute a primitive order, though exhibiting some remarkable adaptive features. This order possibly belongs to the Merostomata, but is distinctly allied to the crustaceans in such important characters as the structure of the legs and telson, and is therefore much generalized.
The specialization of Sidneyia consists in the remarkable development of a highly complex claw on each of the third cephalic appendages, and in the compound tail-fin, built up of the last segment and one or more pairs of swimmerets. These two characteristics seem to preclude the possibility of deriving the eurypterids from Sidneyia itself, but it seems entirely within reason that they may have been derived from another slightly less specialized member of the same order.
That Sidneyia is descended from any known trilobite seems highly improbable, but that it was descended from the same ancestral stock as the trilobites is, I believe, indicated by the presence of five pairs of appendages on the cephalon and trilobitic legs on the abdomen.
Other so called Merostomata found by Walcott in the Middle Cambrian are the genera Molaria and Habelia, both referred to the Cambrian family Aglaspidæ. These genera seem to conform with Aglaspis of the Upper Cambrian in having a trilobite-like cephalon without [121] facial sutures, a trilobite-like thorax of a small but variable (7-12) number of segments, and a Limulus-like telson. Neither of them has yet been fully described or figured, but (Walcott 1912 A, p. 202) Habelia appears to have five pairs of cephalic appendages, the first two pairs of which are multisegmented antennæ. The thoracic appendages are likewise none too well known, but they appear to have been biramous. The endopodites are better preserved than the exopodites, but in at least one specimen of Molaria the exopodites are conspicuous.
If these genera are properly described and figured, their appendages are typically crustacean, and fundamentally in agreement with those of Marrella. The relation to the Trilobita is evidently close, the principal differences being the absence of facial sutures and the presence of true antennæ. I am therefore transferring the Aglaspidæ from the Merostomata to a new subclass under the Crustacea.
The spiders have the head and thorax fused, the abdomen unsegmented except in the most primitive suborder, and so appear even less trilobite-like than the insects. The appendages likewise are highly specialized. The cephalothorax bears six pairs of appendages, the first of which are the pre-oral cheliceræ, while behind the mouth are the pedipalpi and four pairs of ambulatory legs. The posterior pairs of walking legs belong to the thorax, but the anterior ones are to be homologized with the maxillæ of Crustacea, so that the spiders are like the trilobites in having functional walking legs on the head.
The chief likenesses are, however, seen in the very young. On the germ band there appear a pair of buds in front of the rudiments of the cheliceræ which later unite to form the rostrum of the adult. At the time these buds appear, the cheliceræ are post-oral, but afterward move forward so that both rostrum and cheliceræ are in front of the mouth. The rostrum is therefore the product of the union of the antennules, and the cheliceræ are to be homologized with the antennæ. There seems to be some doubt about the homology of the pedipalps with the mandibles, as at least one investigator claims to have found rudiments of a segment between the one bearing the cheliceræ and that with the pedipalps.
Jaworowski (Zool. Anzeiger, 1891, p. 173, fig. 4) has figured the pedipalp from the germ band of Trochosa singoriensis, and called attention to the fact that it consists of a coxopodite and two segmented branches which may be interpreted as exopodite and endopodite. He designated as exopodite the longer branch which persists in the adult, but since the ambulatory legs of Crustacea are endopodites, that would seem a more likely interpretation. As the figure is drawn, the so called endopodite would appear to spring from the proximal segment of the "exopodite." If the two terms were interchanged, the homology with the limb of the trilobite or other crustacean would be quite perfect.
In the young, the abdomen is segmented and the anterior segments develop limb-buds, the first pair of which become the lung books and the last two pairs the spinnerets of the adult. There seems to be some question about the number of segments. Montgomery (Jour. Morphology, vol. 20, 1909, p. 337). reviewing the literature, finds that from eight to twelve have been seen in front of the anal segment. The number seem to vary with the species studied. This of course suggests connection with the anomomeristic trilobites.
The oldest true spiders are found in the Pennsylvanian, and several genera are now known. The head and thorax are fused completely, but the abdomen is distinctly segmented. [122] Some of the Anthracomarti resemble the trilobites more closely than do the Araneæ, as they lack the constriction between the cephalothorax and abdomen. The spiders of the Pennsylvanian have this constriction less perfectly developed than do modern Araneæ, and occupy an intermediate position in this respect. In the Anthracomarti, the pedipalpi are simple, pediform, and all the appendages have very much the appearance of the coxopodites and endopodites of trilobites. Cheliceræ are not known, and pleural lobes are well developed in this group. Anthracomarti have not yet been found in strata older than the Pennsylvanian, but they seem to be to a certain extent intermediate between true spiders and the marine arachnid.
Insecta.
Handlirsch (in several papers, most of which are collected in "Die Fossilen Insekten," 1908) has attempted to show that all the Arthropoda can be derived from the Trilobita, and has advocated the view that the Insecta sprang directly from that group, without the intervention of other tracheate stock. At first sight, this transformation seems almost an impossibility, and the view does not seem to have gained any great headway among entomologists in the fourteen years since it was first promulgated. If an adult trilobite be compared with an adult modern insect, few likenesses will be seen, but when the trilobite is stripped of its specializations and compared with the germ-band of a primitive insect, the theory begins to seem more possible.
Handlirsch really presented very little specific evidence in favor of his theory. In fact, one gets the impression that he has insisted on only two points. Firstly, that the most ancient known insects, the Palæodictyoptera, were amphibious, and their larvæ, which lived in water, were very like the adult. Secondly, that the wings of the Palæodictyoptera probably worked vertically only, and the two main wings were homologous with rudimentary wing-like outgrowths on each segment of the body. These outgrowths have the appearance of, and might have been derived from, the pleural lobes of trilobites.
He figured (1908, p. 1305, fig. 7) a reconstructed larva of a palæodictyopterid as having biramous limbs on each segment, but so far as I can find, this figure is purely schematic, for there seems to be no illustration or description of any such larva in the body of his work.
That the insects arose directly from aquatic animals is of course possible, and Handlirsch's first argument has considerable force. It may, however, be purely a chance that the oldest insects now known to us happen to be an amphibious tribe. The Palæodictyoptera are not yet known to antedate the Pennsylvanian, but there can be no doubt that, insects existed long before that time, and the fact that their remains have not been found is good evidence that the pre-Pennsylvanian insects were not aquatic. Comstock, who has recently investigated the matter, does not believe that the Palæodictyoptera were amphibious (The Wings of Insects, Ithaca, N. Y., 1918, p. 91).
The second argument, that wings arose from the pleural lobes of trilobites, is exceedingly weak. Where most fully set forth (1907, p. 157), he suggests that trilobites may occasionally have left the water, climbed a steep bank or a plant, and then glided back into their native element, taking advantage of the broad flat shape to make a comfortable and gentle descent! This sport apparently became so engaging that the animal tried experiments with flexible wing tips, eventually got the whole of the pleural lobes in a flexible condition, and selected those of the second and third thoracic segments for preservation, while discarding the remainder. The pleural lobes of trilobites are not only too firmly joined to the axial portion [123] of the test to be easily transformed into movable organs, but they are structurally too unlike the veined wings of insects to make the suggestion of this derivation even worthy of consideration.
Tothill (1916) has recently reinvestigated the possible connection between insects, chilopods, and trilobites, and, from the early appearance of the spiracles in the young, came to the conclusion that the insects were derived from terrestrial animals. He suggested that they may have come through the chilopods from the trilobites. The hypothetical ancestor of the insects, as restored by Tothill from the evidence of embryology and comparative anatomy, is an animal more easily derived from the Chilopoda than from the Trilobita. Five pairs of appendages are present on the head, and the trunk is made up of fourteen similar segments, each with a pair of walking limbs and a pair of spiracles.
Only the maxillæ and maxillulæ are represented as biramous. If the ancestor of the Insecta was, as seems possible, tracheate, this fact alone would rule out the trilobites. Among tracheates, the Chilopoda are certainly more closely allied to the Insecta than are any other wingless forms. If the ancestors of the insects were not actually chilopods, they may have been chilopod-like, and there can be little doubt that both groups trace to the same stock.
As to the ancestry of the Chilopoda, it is probable that they had the same origin as the other Arthropoda. Tothill has pointed out that in the embryo of some chilopods there are rudiments of two pairs of antennæ and that the two pairs of maxillæ and the maxillipeds are biramous. This would point rather to the Haplopoda than directly to the trilobites as possible ancestors, and may explain why the former vanish so suddenly from the geological record after their brief appearance in the Middle Cambrian. They may have gone on to the land.
There seem to be no insuperable obstacles to prevent the derivation, indirectly, of the insects from some trilobite with numerous free segments, and small pygidium. The antennules and pleural lobes must be lost, the antennas and trunk limbs modified by loss of exopodites. Wings and tracheæ must be acquired.
Handlirsch places the date of origin of the Insecta rather late, just at the end of the Devonian and during the "Carboniferous." By that time most families of trilobites had died out, so that the possibilities of origin of new stocks were much diminished. If the haplopod-chilopod-insect line is a better approximation to the truth, then the divergence began in the Cambrian.
Chilopoda.
The adult chilopod lacks the antennules, and all of the other appendages, with the exception of the maxillulæ, are uniramous. The walking legs are similar to the endopodites of trilobites, and usually have six or seven segments. The appendages are therefore such as could be derived by modification of those of trilobites by the almost complete loss of the exopodites and shortening of the endopodites of the head. The position of the post-oral appendages, the posterior ones outside those closest the mouth, is perhaps foreshadowed in the arrangement of those of Triarthrus.
The Chilopoda differ from the Hexapoda in developing the antennæ instead of the antennules as tactile organs, but this can not be used with any great effect as an argument that the latter did not arise from the ancestors of the former, since it is entirely possible that in early Palæozoic times the pre-Chilopoda possessed two pairs of antennæ. The first pair are still recognizable in the embryo of certain species.
The oldest chilopods are species described by Scudder (Mem. Boston Soc. Nat. Hist., vol. 4, 1890, p. 417, pl. 38) from the Pennsylvanian at Mazon Creek, Grundy County, Illinois. Only one of these, Latzelia primordialis Scudder (pl. 38 fig. 3), is at all well preserved. This little animal, less than an inch long, had a depressed body, with a median carina, exceedingly long slender legs, and about nineteen segments. The head is very nearly obliterated.
Diplopoda.
The diplopods, especially the polydesmids with their lateral outgrowths, often have a general appearance somewhat like that of a trilobite, but on closer examination few likenesses are seen. The most striking single feature of the group, the possession by each segment of two pairs of appendages, is not in any way foreshadowed in the trilobites, none of which shows any tendency toward a fusion of pairs of adjacent segments. The antennules are short, antennæ absent, mandibles and maxillulæ much modified, the latter possibly biramous, and the maxillæ absent. The trunk appendages are very similar to those of chilopods, and could readily be derived from the endopodites of trilobites.
The oldest diplopods are found in the Silurian (Ludlow) and Devonian (Lower Old Red) of Scotland, and three species belonging to two genera are known. The oldest is Archidesmus loganensis Peach (1889, p. 123, pl. 4, fig. 4), and the Devonian species are Archidesmus macnicoli Peach and Kampecaris forfarensis Page (Peach 1882, p. 182, pl. 2, fig. 2, 2a, and p. 179, pl. 2, figs. 1-1g). All of these species show lateral expansions like the recent Polydesmidæ, and these of course suggest the pleural lobes of trilobites. All three of the species are simpler than any modern diplopod, for there is only a single pair of appendages on each segment. No foramina repugnatoria were observed, and the eyes of Kampecaris forfarensis as described are singularly like those of a phacopid.
Peach says: "The eye itself is made up of numerous facets which are arranged in oblique rows, the posterior end of each row being inclined downwards and outwards, the facets being so numerous and so close together that the eye simulates a compound one." There is also a protecting ridge which somewhat resembles a palpebral lobe (1882, pl. 7, fig. la). Peach comments on the strength of the test, and from his description it appears that it must have been preserved in the same manner as the test of trilobites. It was punctate, and granules and spines were also present. The presence of the lateral outgrowths in these ancient specimens would seem to indicate that they are primitive features, and may have been inherited. While possibly not homologous with the pleural extensions of trilobites, they may be vestiges of these structures.
The limbs are made up of seven segments which are circular in section and expand at the distal end. The distal one bears one or two minute spines. They are most readily compared with the endopodites of Isotelus. The resemblance is, in fact, rather close. The sternal plates are wider and the limbs of opposite sides further apart than in modern diplopods. Except for one pair of antennæ, no cephalic appendages are preserved.
While these specimens do not serve to connect the Diplopoda with the Trilobita, they do show that most of the specializations of the former originated since Lower Devonian times, and lead one to suspect that the derivation from marine ancestors took place very early, perhaps in the Cambrian. If no very close connection with the trilobites is indicated, there is also nothing to show that the diplopods could not have been derived from that group.
Primitive Characteristics of Trilobites.
TRILOBITES THE MOST PRIMITIVE ARTHROPODS.
The Arthropoda, to make the simplest possible definition, are invertebrate animals with segmented body and appendages. The most primitive arthropod would appear to be one composed of exactly similar segments bearing exactly similar appendages, the segments of the appendages themselves all similar to one another. It is highly improbable that this most primitive arthropod imaginable will ever be found, but after a survey of the whole phylum, it appears that the simpler trilobites approximate it most closely.
That the trilobites are primitive is evidenced by the facts that they have been placed at the bottom of the Crustacea by all authors and claimed as the ancestors of that group by some; that Lankester derived the Arachnida from them; and that Handlirsch has considered them the progenitors of the whole arthropodan phylum.
Specializations among the Arthropoda, even among the free-living forms, are so numerous that it would be difficult to make a complete list of them. In discussing the principal groups, I have tried to show that the essential structures can be explained as inherited from the Trilobita, changed in form by explainable modifications, and that new structures, not' present in the Trilobita, are of such a nature that they might be acquired independently in even unrelated groups.
The chief objections to the derivation of the remainder of the Crustacea from the trilobites have been: first, that the trilobites had broad pleural extensions; second, that they had a large pygidium; and lastly, that they had only one pair of tactile antennæ.
It has now been pointed out that many modern Crustacea have pleural extensions, but that they usually bend down at the sides of the body, and also that in the trilobites and more especially in Marrella, there was a tendency toward the degeneration of the pleural lobes. A glance at the Mesonacidæ or Paradoxidæ should be convincing proof that in some trilobites the pygidium is reduced to a very small plate.
In regard to the second antennæ standard text-books contain statements which are actually surprising. A compilation shows that the antennæ are entirely uniramous in but a very few suborders, chiefly among the Malacostraca; that they are biramous with both exopodite and endopodite well developed in most Copepoda, Ostracoda, and Branchiopoda; and that the exopodite, although reduced in size, still has a function in some suborders of the Malacostraca. The Crustacea could not possibly be derived from an ancestor with two pairs of uniramous antennæ.
Although I have defended the trilobites, perhaps with some warmth, from the imputation that they were Arachnida, my argument does not apply in the opposite direction, and I believe Lankester was right in deriving the Arachnida from them. If the number of appendages in front of the mouth is fundamental, then the trilobites were generalized, primitive, and capable of giving rise to both' Crustacea and Arachnida. As shown on a previous page (p. 119), the "connecting links" so far found tend to disprove rather than to prove the thesis, but the present finds should be looked upon as only the harbingers of the greater ones which are sure to come.
LIMBS OF TRILOBITES PRIMITIVE.
The general presence, in an adult or larva, of some sort of biramous limbs throughout the whole class Crustacea has led most zoologists to expect such a limb in the most [126] primitive crustaceans, and apparently the appendage of the trilobite satisfies the expectation. It is well, perhaps, as a test, to consider whether by modification this limb could produce the various types of limbs seen in other members of the class. In the first place, it is necessary to have clearly in mind the peculiarities of the appendage to be discussed.
It should first of all be remembered that the limb is articulated with the dorsal skeleton in a manner which is very peculiar for a crustacean. The coxopodite swings on a sort of ball-and-socket joint, and at the outer end both the exopodite and the basipodite articulate with it. Since the exopodite articulates with the basipodite as well as with the coxopodite, the two branches are closely connected with one another and there is little individual freedom of movement. This is, of course, a necessary consequence of their articulation with a segment which is itself too freely movable to provide a solid base for attachment of muscles. The relation of the appendifer, coxopodite, and two rami is here shown diagrammatically (fig. 33), the exopodite branching off from the proximal end of the basipodite at the junction with the coxopodite.
In all trilobites the endopodite consists of six segments, and the coxopodite of a single segment the inner end of which is prolonged as an endobase. There does not seem to be any variation from this plan in the subclass, although individual segments are variously modified. The exopodites are more variable, but all consist of a flattened shaft with setæ on one margin. No other organs such as accessory gills, swimming plates, or brood pouches have yet been found attached to the appendages, the evidence for the existence of the various epipodites and exites described by Walcott being unsatisfactory (see p. 23).
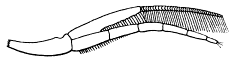
Fig. 33.—Diagrammatic representation of an appendage of the anterior end of the thorax of Triarthrus becki Green, to show relation of exopodite and endopodite to each other and to the coxopodite. Much enlarged.
In the Ostracoda the appendages are highly variable, but it is easily seen that they are modifications of a limb which is fundamentally biramous. In most species, both exopodite and endopodite suffer reduction. The exopodite springs from the basipodite and that segment is closely joined to the coxopodite, producing a protopodite. In some cases the original segments of the endopodites fuse to form a stiff rod. While highly diversified, these appendages are very trilobite-like, and some Ostracoda even have biramous antennæ.
The non-parasitic Copepoda have limbs exceedingly like those of trilobites. Many of them are biramous, the endopodites sometimes retaining the primitive six segments. Coxopodite and basipodite are generally united, and endopodite and exopodite variously modified. Like some of the Ostracoda, the more primitive Copepoda have biramous antennæ.
As would be expected, the appendages of the Cirripedia are much modified, although those of the nauplius are typical. The thoracic appendages of many are biramous, but both branches are multisegmented.
In the modern Malacostraca the ground plan of the appendages is biramous, but in most orders they are much modified. In many, however, the appendages of some part of the body are biramous, and in many the endopodites show the typical six segments. From the coxopodites arise epipodites, some of which assist in swimming, and some in respiration. [127] Because of the many instances in which such extra growths arise, and because of the form of the appendages of the Branchiopoda, it has been suggested that the primitive crustacean leg must have been more complex than that of the trilobite. In looking over the Malacostraca, however, one is struck by the fact that epipodites generally arise where the exopodites have become aborted or are poorly developed, and seem largely to replace them. The coxopodite and basipodite are usually fused to form a protopodite, and a third segment is sometimes present in the proximal part of the appendage.
In the Branchiopoda are found the most complex crustacean limbs, and the ones most difficult to homologize with those of trilobites. In recent years, Lankester's homologies of the parts of the limbs of Apus with those of the Malacostraca have been quite generally accepted, and the appendages of the former considered primitive. Now that it is known that the Branchiopoda of the Middle Cambrian (Burgessia et at.) had simple trilobite-like appendages, it becomes necessary to exactly reverse the opinion in this matter. The same homologies stand, but the thoracic limbs of Apus must be looked upon as highly specialized instead of primitive.
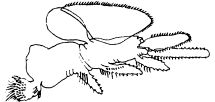
Fig. 34.—One of the appendages of the anterior part of the trunk of Apus, showing the endites (beneath) and exites (above). The proximal endite forms a gnathobase which is not homologous with the gnathobase (or endobase) of the trilobite. Copied from Lankester. Much enlarged.
Lankester (Jour. Micros. Sci., vol. 21, 1881) pointed out that the axial part of the thoracic limb of Apus (fig. 34) is homologous with the protopodite in the higher Crustacea, that the two terminal endites corresponded to the exopodite and endopodite, and that the other endites and exites were outgrowths from the protopodite analogous to the epipodites of Malacostraca. There seems to be no objection to retaining this interpretation, but with the meaning that both endopodite and exopodite are much reduced, and their functions transferred to numerous outgrowths of the protopodite. One of the endites grows inward to form an endobase, the whole limb showing an attempt to return to the ancestral condition of the trilobite. The limbs of some other branchiopods are not so easy to understand, but students of the Crustacea seem to have worked out a fairly satisfactory comparison between them and Apus.
The discovery that the ancestral Branchiopoda had simple biramous appendages instead of the rather complex phyllopodan type is another case in which the theory of "recapitulation" has proved to hold. It had already been observed that in ontogeny the biramous limb preceded the phyllopodan, but so strong has been the belief in the primitive character of the Apodidæ that the obvious suggestion has been ignored. Even in such highly specialized Malacostraca as the hermit crabs the development of certain of the limbs illustrates the change from the schizopodal to the phyllopodan type, and Thompson (Proc. Boston Soc. Nat. Hist., vol. 31, 1903, pl. 5, fig. 12) has published an especially good series of drawings [128] showing the first maxilliped. In the first to fourth zoeæ the limb is biramous but in the glaucothoe a pair of broad processes grow out from the protopodite, while the exopodite and particularly the endopodite become greatly reduced. In the adult the endopodite is a mere vestige, while the flat outgrowths from the protopodite have become very large and bear setæ.
The limbs of most Crustacea are readily explained as modifications of a simple biramous type. These modifications usually take the form of reduction by the loss or fusion of segments and quite generally either the entire endopodite or exopodite is lacking. Modification by addition frequently occurs in the growth of epipodites, "endites," and "exites" from the coxopodite, basipodite, or both. A protopodite is generally formed by the fusion of coxopodite and basipodite, accompanied by a transference of the proximal end of the exopodite to the distal end of the basipodite. A new segment, not known in the trilobites (precoxal), is sometimes added at the inner end.
Among modern Crustacea, the anterior cephalic appendages and thoracic appendages of the Copepoda and the thoracic appendages of certain Malacostraca, Syncarida especially, are most nearly like those of the trilobite. The exact homology, segment for segment, between the walking legs of the trilobite and those of many of the Malacostraca, even the Decapoda, is a striking instance of retention of primitive characteristics in a specialized group, comparable to the retention of primitive appendages in man.
NUMBER OF SEGMENTS IN THE TRUNK.
Various attempts have been made to show that despite the great variability, trilobites do show a tendency toward a definite number of segments in the body.
Emmrich (1839), noting that those trilobites which had a long thorax usually had a short pygidium, and that the reverse also held true, formulated the law that the number of segments in the trunk was constant (20 + 1) Very numerous exceptions to this law were, however, soon discovered, and while the condition of those with less than twenty-one segments was easily explained, the increasing number of those with more than twenty-one soon brought the idea into total disrepute.
Quenstedt (1837) had considered the number of segments of at least specific importance, and both he and Burmeister (1843) considered that the number of segments in the thorax must be the same for all members of a genus. As first shown by Barrande (1852. p. 191 et seq.), there are very many genera in which there is considerable variation in the number of thoracic segments, and a few examples can be cited in which there is variation within a species, or at least in very closely related species.
Carpenter (1903, p. 333) has tabulated the number of trunk segments of such trilobites as were listed by Zittel in 1887 and finds a steady increase throughout the Palæozoic. His table, which follows, is, however, based upon very few genera.
Period | No. of Genera |
Average No. of body-segments |
Cambrian | 12 | 17.66 |
Ordovician | 23 | 18.58 |
Silurian | 16 | 19.34 |
Devonian | 10 | 20.70 |
Carboniferous | 02 | 20.75 |
Due chiefly to the efforts of Walcott, an increasingly large number of Cambrian genera are now represented by entire specimens, and since these most ancient genera are of greatest importance, a few comments on them may be offered.
The total number of segments can be fairly accurately determined in at least nineteen genera of trilobites from the Lower Cambrian. These include eight genera of the Mesonacidæ (Olenellus was excluded) and Eodiscus, Goniodiscus, Protypus, Bathynotus, Atops, Olenopsis, Crepicephalus, Vanuxemella, Corynexochus, Bathyuriscus, and Poliella. The extremes of range in total segments of the trunk is seen in Eodiscus (9) and Pædeumias (45+), and these same genera show the extremes in the number of thoracic segments, there being 3 in the one and 44+ in the other. Pædeumias probably shows the greatest variation of any one genus of trilobites, various species showing from 19 to 44+ thoracic segments. The average for the nineteen genera is 13.9 segments in the thorax, 3.7 segments in the pygidium, or a total average of 17.6 segments in the trunk. Crepicephalus with 12-14 segments in the thorax and 4-6 in the pygidium, and Protypus, with 13 in the thorax and 4-6 in the pygidium, are the only genera which approach the average. All of the Mesonacidæ, except one, Olenelloides, have far more thoracic and fewer pygidial segments than the average, while the reverse is true of the Eodiscidæ, Vanuxemella, Corynexochus, Bathyuriscus, and Poliella.
The eight genera of the Mesonacidæ, Nevadia, Mesonacis, Elliptocephala, Callavia, Holmia, Wanneria, Pædeumias, and Olenelloides, have an average of 20.25 segments in the thorax and 1.5 in the pygidium, a total of 21.75. If, however, the curious little Olenelloides be omitted, the average for the thorax rises to 22.14 and the total to 23.84. Olenelloides is, in fact, very probably the young of an Olenellus. Specimens are only 4.5 to 11 mm. long, and occur in the same strata with Olenellus (see Beecher 1897 A, p. 191).
Thirty-three genera from the Middle Cambrian afford data as to the number of segments, the Agnostidæ being excluded. The extreme of variation there is smaller than in the Lower Cambrian. The number of thoracic segments varies from 2 in Pagetia to 25 in Acrocephalites, and these same genera show the greatest range in total number of trunk segments, 8 and 29 respectively.
The average of thoracic segments for the entire thirty-three genera is 10.5, of pygidial segments 5.9, a total average of 16.4. It will be noted that the thorax shows on the average less and the pygidium more segments than in the Lower Cambrian. If the Agnostidæ could be included, this result would doubtless be still more striking. Of the genera considered, Asaphiscus with 7-11 thoracic and 5-8 pygidial segments, Blainia with 9 thoracic and 6-11 pygidial, Zacanthoides with 9 thoracic and 5 pygidial, and Anomocare with 11 thoracic and 7-8 pygidial segments came nearest to the average. Only a few departed widely from it. The genera tabulated were Acrocephalites, Alokistocare, Crepicephalus, Karlia, Hamburgia, Corynexochus, Bathyuriscus, Poliella, Agraulos, Dolichometopus, Ogygopsis, Orria, Asaphiscus, Neolenus, Burlingia, Blainia, Blountia, Marjumia, Pagetia, Eodiscus, Goniodiscus, Albertella, Oryctocara, Zacanthoides, Anomocare, Anomocarella, Coosia, Conocoryphe, Ctenocephalus, Paradoxides, Ptychoparia, Sao, and Ellipsocephalus.
Enough genera of Upper Cambrian trilobites are not known from entire specimens to furnish satisfactory data. Excluding from the list the Proparia recently described by Walcott, the average total trunk segments in ten genera is 18, but as most of the genera are Olenidæ or olenid-like, not much weight can be attached to these figures.
For the Cambrian as a whole, the average for sixty-two genera is between 17 and 18 trunk segments, which is surprisingly like the result obtained by Carpenter from only twelve [130] genera, and tends to indicate that it must be somewhere near the real average. If the 5 or 6 segments of the head be added, it appears that the "average" number of segments is very close to the malacostracan number 21. Genera with 16 to 18 trunk segments are Callavia, Protypus, Bathynotus, Crepicephalus, Bathyuriscus, Ogygopsis, Burlingia, Orria, Asaphiscus, Blainia, Zacanthoides, Neolenus, Anomocare, Conocoryphe, Saukia, Olenus, and Eurycare.
The order Proparia originated in the Cambrian, and Walcott has described four genera, one from the Middle, and three from the Upper. The number of segments in these genera is of interest. Burlingia, the oldest, has 14 segments in the thorax and 1 in the pygidium. Of the three genera in the Upper Cambrian, Norwoodia has 8-9 segments in the thorax and 3-4 in the pygidium; Millardia 23 in thorax and 3-4 in pygidium; and Menomonia 42 in thorax and 3-4 in pygidium. It is of considerable interest and importance to note that the very elongate ones are not from the Middle but from the Upper Cambrian.
Forty genera of Ordovician trilobites known from entire specimens were tabulated, and it was found that the range in the number of segments in the thorax and pygidium was surprisingly large. Agnostus, which was not included in the table, has the fewest, and Eoharpes, with 29, the most. While the range in number of segments in the thorax is 2 to 29, the range of the number in the pygidium, 2 to 26, is almost as great. A species of Dionide has 26 in the pygidium, while Remopleurides and Glaphurus have evidence of only 2. The average number of segments in the thorax for the forty genera was 10.15, in the pygidium 8.81, and the average number for the trunk 19.
Genera with just 19 segments in the trunk appear to be rare in the Ordovician, a species of Ampyx being the only one I have happened to notice. Calymene, Tretaspis, Triarthrus, Asaphus, Ogygites, and Goldius come with the range of 18 to 20. Goldius, with 10 segments in the thorax and (apparently) 8 in the pygidium, comes nearest to the averages for these two parts of the trunk. Goldius, Amphilichas, Bumastus, Acidaspis, Actinopeltis, and Sphærexochus are among the genera having 10 segments in the thorax, and there are many genera which have only one or two segments more or less than 10.
In most Ordovician genera, thirty-five out of the forty tabulated, the number of segments in the thorax is fixed, and the variation is in any case small. In four of the five genera where it was not fixed, there was a variation of only one segment, and the greatest variation was in Pliomerops, where the number is from 15 to 19. This of course indicates that the number of segments in the thorax tends to become fixed in Ordovician time. The variation in the number of segments in the pygidium is, however, considerable. It is difficult in many cases to tell how many segments are actually present in this shield, as it is more or less smooth in a considerable number of genera. Extreme cases of variation within a genus are found in Encrinurus, species of which have from 7 to 22 segments in the pygidium, Cybeloides with 10 to 20, and Dionide with 10 to 26. As the number in the thorax became settled, the number in the pygidium became more unstable, so that not even in the Ordovician can the total number of segments in the trunk be said to show any tendency to become fixed.
The genera used in this tabulation were: Eoharpes, Cryptolithus, Tretaspis, Trinucleus, Dionide, Raphiophorus, Ampyx, Endymionia, Anisonotus, Triarthrus, Remopleurides, Bathyurus, Bathyurellus, Ogygiocaris, Asaphus, Ogygites, Isotelus, Goldius, Cyclopyge, Amphilichas, Odontopleura, Acidaspis, Glaphurus, Encrinurus, Cybele, Cybeloides, Ectenonotus, Calymene, Ceraurus, Pliomera, Pliomerops, Pterygometopus, Chasmops, Eccoptochile, Actinopeltis, Sphærexochus, Placoparia, Pilekia, Selenopeltis, and Calocalymene.
Only sixteen genera of Devonian trilobites were available for tabulation, and it is not always possible to ascertain the exact number of segments in the pygidium, although genera with smooth caudal shields had nearly all disappeared. The number of segments in the thorax had become pretty well fixed by the beginning of the Devonian, Cyphaspis with a range of from 10 to 17 furnishing the only notable exception. The range for the sixteen genera is from 8 to 17, the average 11, the number exhibited by the Phacopidæ which form so large a part of the trilobites of the Devonian. The greater part of the species have large pygidia, and while the range is from 3 to 23, the average is 11.2. Probolium, with 11 in the thorax and 11-13 in the pygidium, and Phacops, with 11 in the thorax and 9-12 in the pygidium, approach very closely to the "average" trilobite, and various species of other genera of the Phacopidæ have the same number of segments as the norm. In every genus, however, the number of segments in the pygidium is variable, the greatest variation being in Dalmanites, with a range of from 9 to 23. The number of segments in the pygidium was therefore not fixed and was on the average higher than in earlier periods.
The genera used in the tabulation were: Calymene, Dipleura, Goldius, Proëtus, Cyphaspis, Acidaspis, Phacops, Hausmania, Coronura, Odontochile, Pleuracanthus, Calmonia, Pennaia, Dalmanites, Probolium, and Cordania.
The trilobites of the late Palæozoic (Mississippian to Permian) belong, with two possible exceptions, to the Pröetidæ, and only three genera, Proëtus, Phillipsia, and Griffithides, appear to be known from all the parts. I am, however, assuming that both Brachymetopus and Anisopyge have 9 segments in the thorax, and so have tabulated five genera. The range in the number of segments in the pygidium is large, from 10 in some species of Proëtus to 30 in Anisopyge, and the average, 17.3, is high, as is the average for total number in the trunk, 26.3. Anisopyge, a late Permian trilobite described by Girty from Texas, is perhaps the last survivor of the group. It seems to have had 39 segments in the trunk, making it, next to the Cambrian Pædeumias and Menomonia, the most numerously segmented of all the trilobites.
The above data may be summarized in the following table:
Period | No. of genera |
Av. No. of segments in thorax |
Av. No. of segments in pygidium |
Av. No. of trunk segments |
Lower Cambrian | 19 | 13.90 | 03.70 | 17.60 |
Middle Cambrian | 33 | 10.50 | 05.90 | 16.40 |
Entire Cambrian | 62 | ... | ... | 17-19 |
Ordovician | 40 | 10.15 | 08.81 | 18.96 |
Devonian | 16 | 11.00 | 11.20 | 22.20 |
Late Palæozoic | 05 | 09.00 | 17.30 | 26.30 |
This table confirms that made up by Carpenter, and shows even more strikingly the progressive increase in the average number of segments in the trunk throughout the Palæozoic.
While the two trilobites with the greatest number of segments are Cambrian, yet on the average, the last of the trilobites had the more numerously segmented bodies. The multisegmented trilobites are:
Period | Genus | Av. No. of segments in thorax |
Av. No. of segments in pygidium |
Av. No. of trunk segments |
Lower Cambrian | Pædeumias | 44+.... | 01 | 45+ |
Upper Cambrian | Menomonia | 42..... | 04 | 46. |
Ectenonotus | 12..... | 22 | 34. | |
Ordovician | Encrinurus | 11..... | 22 | 33. |
Dionide | 06..... | 26 | 32. | |
Silurian | Harpes | 29..... | 03 | 32. |
Devonian | Coronura | 11..... | 23 | 34. |
Dalmanites | 11..... | 23 | 34. | |
Permian | Anisopyge | 07+(9?) | 30 | 39? |
Anisopyge, the last of the trilobites, stands third on the list of those having great numbers of segments, and in each period there are a few which have considerably more than the average number. It may be of some significance that of these nine genera only Pædeumias and Anisopyge belong to the Opisthoparia, the great central group, and that five are members of the Proparia, the latest and most specialized order.
FORM OF THE SIMPLEST PROTASPIS.
It would naturally be expected that the young of the Cambrian trilobites should be more primitive than the young of species from later formations, and Beecher (1895 C) has shown that this is the case. He had reference, however, chiefly to the eyes, free cheeks, and spines, and by comparison of ontogeny and phylogeny, demonstrated the greater simplicity of the protaspis which lacked these organs. It remains to inquire which among the other characteristics are most fundamental.
Among the trilobites of the Lower Cambrian, no very young have been seen except of Mesonacidæ. Of these, the ontogeny of Elliptocephala asaphoides Emmons is best known, thanks to Ford, Walcott, and Beecher, but, as the last-named has pointed out, the actual protaspis or earliest shield has not yet been found. The youngest specimen is the one roughly figured by Beecher (1895 C, p. 175, fig. 6). It lacks the pygidium, but if completed by a line which is the counterpart of the outline of the cephalon, it would have been 0.766 mm. long. The pygidium would have been 0.183 mm. long, or 23 per cent of the whole length. The axial lobe was narrow, of uniform width along the cephalon, showed a neck-ring and four indistinct annulations, but did not reach quite to the anterior end, there being a margin in front of the glabella about 0.1 mm. wide. The greatest width of the cephalon was 0.66 mm., and of the glabella 0.233 mm., or practically 35 per cent of the total width. Other young Elliptocephala up to a length of 1 mm., and young Pædeumias, Mesonacis, and Holmia (see Kiær, Videnskaps, Skrifter, 1 Mat.-Naturv. Klasse, 1917, No. 10) show about the same characteristics, but all these have large compound eyes on the dorsal surface and specimens in still younger stages are expected. It may be pointed out, however, that in these specimens the pygidium is proportionately larger than in the adult. Walcott cites one adult 126 mm. long in which the pygidium is 6 mm. long, or between 4 and 5 per cent of the total length, while in the incomplete specimen described above, it was apparently 23 per [133] cent. In a specimen 1 mm. long figured by Walcott, the pygidium is 0.15 mm. long, or 15 per cent of the whole length.
The development of several species of trilobites from the Middle Cambrian is known. Barrande (1852) described the protaspis of Sao hirsuta, Peronopsis integer, Phalacroma bibullatum, P. nudum, and Condylopyge rex. Broegger figured that of a Liostracus (Geol. For. Förhandl., 1875, pl. 25, figs. 1-3) and Lindstroem (1901, p. 21) has reproduced the same. Matthew (Trans. Roy. Soc. Canada, vol. 5, 1888, pl. 4, pls. 1, 2) has described the protaspis of a Liostracus, Ptychoparia linnarssoni Broegger, and Solenopleura robbi Hartt. Beecher (1895 C, pl. 8) has figured the protaspis of Ptychoparia kingi Meek, and the writer that of a Paradoxides (Bull. Mus. Comp. Zool., vol. 58, No. 4, 1914, pl. i).
Sao, Liostracus, Ptychoparia, and Solenopleura all have the same sort of protaspis. In all, the axial lobe reaches the anterior margin and is somewhat expanded at that end; in all, the glabella shows but slight trace of segmentation; and in all, the pygidium occupies from one fifth to one fourth the total length. There is considerable variation in the width of the axial lobe. It is narrowest in Ptychoparia, where in the middle it is only 14 per cent of the whole width, and widest in Solenopleura, where it is 28 per cent. In Ptychoparia the pygidium of the protaspis occupies from 18 to 22 per cent of the whole length. In the adult it occupies 10 to 12 per cent. In Solenopleura it makes up about 26 per cent of the protaspis, and in the adult about 8 per cent.
In the youngest stages of all these trilobites, the pygidium is incompletely separated from the cephalon. The first sign of segmentation is a transverse crack which begins to separate the cephalon and pygidium, and by the time this has extended across the full width the neck segment has become rather well defined. In this stage the animal is prepared to swim by means of the pygidium, and first becomes active. The coincident development of the free pygidium and the neck-ring strongly suggests that the dorsal longitudinal muscles are attached beneath the neck-fur row.
The single protaspis of Paradoxides now known, while only 1 mm. long, is not in the youngest stage of development. It is like the protaspis of Olenellus in having large eyes on the dorsal surface and a narrow brim in front of the glabella. The glabella is narrower than in the adult.
The initial test of no agnostid has probably as yet been seen, as all the young now known show the cephalon and pygidium distinctly separated. Phalacroma bibullatum and P. nudum are both practically smooth and isopygous when 1.5 mm. long. P. bibullatum shows no axial lobe at this stage, but a wide glabella and median tubercle develop later, and when the glabella first appears, it extends to the anterior margin. In Peronopsis integer and Condylopyge rex, the axial lobe is outlined on each of the equal shields in specimens about 1 mm. long, but is without furrows and reaches neither anterior nor posterior margin.
From the foregoing brief description it appears that the pygidium of the protaspis varies in different groups from as little as 15 per cent of the total length in the Mesonacidæ to as much as 50 per cent in the Agnostidæ; that the axial lobe varies from as little as 14 per cent of the total width in one Ptychoparia to as much as 50 per cent in Phalacroma nudum; that the glabella reaches the anterior margin in the Olenidæ, Solenopleuridæ, and Phalacroma bibullatum, while there is a brim in front of it in the Olenellidæ, Paradoxidæ, and three of the species of the Agnostidæ. The decision as to which of these conditions are primitive may be settled quite satisfactorily by study of the ontogeny of the various species.
Taking first the pygidium, it has already been pointed out that in each case the pygidium of the adult is proportionally considerably smaller than the pygidium of the protaspis. The stages in the growth of the pygidium are better known in Sao hirsuta than in any other trilobite, and a review of Barrande's description will be advantageous.
Barrande recognized twenty stages in the development of this species, but there was evidently a still simpler protaspis in his hands than the smallest he figured, for he says, after describing the specimen in the first stage: "We possess one specimen on which the head extends from one border to the other of the disk, but as this individual is unique we have not thought it sufficient to establish a separate stage." This specimen is important as indicating a stage in which there was not even a suggestion of division between cephalon and pygidium.
In the first stage described by Barrande, the form is circular, the length is about 0.66 mm., and the glabella is narrow with parallel sides and no indications of lateral furrows. The neck segment is indicated by a slight prominence on the axial lobe, and back of it a constriction divides the axial lobe of the pygidium into two nodes, but does not cross the pleural lobes. The position of the nuchal segment permits a measurement of the part which is to form the pygidium, and shows that that shield made up 30 per cent of the entire length.
In the second stage, when the test is 0.75 mm. long, the cephalon and pygidium become distinctly separated, and the latter shield shows three annulations on the axial and two pairs of ribs on the pleural lobes. It now occupies 33-1/3 per cent of the total length.
In the third stage, when the total length is about 1 mm., the pygidium has continued to grow. It now shows five annulations on the axial lobe, and is 46 per cent of the total length.
In the fourth stage, two segments of the axial lobe have been set free from the front of the pygidium. The length is now 1.5 mm. and the pygidium makes up 32 per cent of the whole. From this time the pygidium continues to decrease in size in proportion to the total length, as shown in the following table.
Stage | Length in mm. |
Percentage of pygidium |
Segments in thorax |
Segments in pygidium |
1 | 0.66 | 30 | 0 | 2 |
2 | 0.75 | 33-1/3 | 0 | 3 |
3 | 1.00 | 46 | 0 | 5 |
4 | 1.50 | 32 | 2 | 5-6 |
5 | 1.50 | 25 | 3 | 4 |
6 | 1.75 | 23 | 4 | 4 |
7 | 1.80 | 21 | 5 | 3 |
8 | 2.00 | 17 | 6 | 3 |
9 | 2.50 | 13 | 7 | 3 |
10 | 3.00 | 12 | 8 | 3 |
11 | 3.50 | 11 | 9 | 3-4 |
12 | 4.00 | 11 | 10 | 3-4 |
13 | 5.00 | 10 | 11 | 3 |
14 | 5.50 | 9 | 12 | 2-4 |
15 | 6.00 | 8 | 13 | 3-4 |
16 | 6.50 | 8 | 14 | 3 |
17 | 7.00 | 7 | 15 | 3 |
18 | 7.50 | 7 | 16 | 3 |
19 | 7.50 | 6 | 17 | 2 |
20 | 10.25 | 6 | 17 | 2 |
This table shows the rapid increase in the length of the pygidium till the time when the thorax began to be freed, the very rapid decrease during the earlier part of its formation until six segments had been set free, and then a more gradual decrease until the entire seventeen segments had been acquired, after which time the relative length remained constant. From an initial proportion of 30 per cent, it rose to nearly one half the whole length, and then dwindled to a mere 6 per cent, showing conclusively that the thorax grew at the expense of the pygidium.
If this conclusion can be sustained by other trilobites, it indicates that the large pygidium is a more primitive characteristic of a protaspis than is a small one. I have already shown that the pygidium is proportionately larger in the protaspis in the Mesonacidæ, Solenopleuridæ, and Olenidæ, and a glance at Barrande's figures of "Hydrocephalus" carens and "H." saturnoides, both young of Paradoxides will show that the same process of development goes on in that genus as in Sao. There is first an enlargement of the pygidium to a maximum, a rise from 20 per cent to 33 per cent in the case of H. carens and then, with the introduction of thoracic segments, a very rapid falling off. All of these are, however, trilobites with small pygidia, and it has been a sort of axiom among palæontologists that large pygidia were made up of a number of coalesced segments. While not definitely so stated, it has generally been taken to mean the joining together of segments once free. The asaphid, for instance, has been thought of as descended from some trilobite with rich segmentation, and a body-form like that of a Mesonacis or Paradoxides.
The appeal to the ontogeny does not give as full an answer to this question as could be wished, for the complete life-history of no trilobite with a large pygidium is yet known. While the answer is not complete, enough can be gained from the study of the ontogeny of Dalmanites and Cyclopyge to show that in these genera also the thorax grows by the breaking down of the pygidium and that no segment is ever added from the thorax to the pygidium. The case of Dalmanites socialis as described by Barrande (1852, p. 552, pl. 26) will be taken up first, as the more complete. The youngest specimen of this species yet found is 0.75 mm. long, the pygidium is distinctly separated from the cephalon, and makes up 25 per cent of the length. This is probably not the form of the shell as it leaves the egg. At this stage there are two segments in the pygidium, but they increase to four when the test is 1 mm. long. The cephalon has also increased in length, however, so that the proportional length is the same. The subjoined table, which is that compiled by Barrande with the proportional length of the pygidium added, is not as complete as could be desired, but affords a very interesting history of the growth of the caudal shield. The maximum proportional length is reached before the introduction of thoracic segments, and during the appearance of the first five segments the size of the pygidium drops from 25 to 15 per cent. Several stages are missing at the critical time between stages 8 and 9 when the pygidium had added three segments to itself and has supplied only one to the thorax. This would appear to have been a sort of resting or recuperative stage for the pygidium, for it increased its own length to 20 per cent, but from this stage up to stage 12 it continued to give up segments to the thorax and lose in length itself. After stage 12, when the specimens were 8 mm. long, no more thoracic segments were added, but new ones were introduced into the pygidium, until it reached a size equal to one fifth the entire length, as compared with one fourth in the protaspis.
Stage | Length in mm. |
Percentage of pygidium |
Segments in thorax |
Segments in pygidium |
1 | 0.75 | 25 | 0 | 2 |
2 | 0.75 | 25 | 0 | 3 |
3 | 1.00 | 25 | 0 | 4 |
4 | 1.00 | 22 | 1 | 3 |
5 | 1.25 | 20 | 2 | 3 |
6 | 1.25 | 18 | 3 | 3 |
7 | 1.60 | 15 | 4 | 3 |
8 | 1.60 | 15 | 5 | 3 |
9 | 3.00 | 20 | 6 | 6 |
10 | 3.50 | 20 | 7 | 6 |
11 | 8.00 | 18 | 9 | 7 |
12 | 8.00 | 16 | 11 | 5 |
13 | 12.00 | 16 | 11 | 7 |
14 | 19.00 | 18 | 11 | 9 |
15 | 95.00 | 20 | 11 | 11 |
Since the above was written, Troedsson (1918, p. 57) has described the development of Dalmanites eucentrus, a species found in the Brachiopod shales (Upper Ordovician) of southern Sweden. This species follows a course similar to that of D. socialis, so that the full series of stages need not be described. The pygidium is, however, of especial interest, for there is a stage in which it shows two more segments than in the adult. Troedsson figures a pygidium 1.28 mm. long which has eight pairs of pleural ribs, while the adult has only six pairs. The ends of all these ribs are free spines, and were the development not known one would say that this was a case of incipient fusion, while as a matter of fact, it is incipient freedom.
A further interest attaches to this case, because of the close relationship between D. eucentrus and D. mucronatus. The latter species appears first in the Staurocephalus beds which underlie the Brachiopod shales, so that in its first appearance it is somewhat the older. The pygidium of the adult D. mucronatus is larger than that of D. eucentrus, having eight pairs of pleural ribs, the same number as in the young of the latter. In short, D. eucentrus is probably descended from D. mucronatus, and in its youth passes through a stage in which it has a large pygidium like that species. Once more it appears that the small pygidium is more specialized than the large one.
The full ontogeny of Cyclopyge is not known, but young specimens show conclusively that segments are not transferred from the thorax to the pygidium, but that the opposite occurs. As shown by Barrande (1852) and corroborated by specimens in the Museum of Comparative Zoology, the process is as follows: The third segment of the adult of this species, that is, the fourth from the pygidium, bears a pair of conspicuous cavities on the axial portion. In a young specimen, 7 mm. long, the second segment bears these cavities, but as the thorax has only four segments, this segment is also the second instead of the fourth ahead of the pygidium. The pygidium itself, instead of being entirely smooth, as in the adult state, is smooth on the posterior half, but on the anterior portion has two well formed but still connected segments, the anterior one being more perfect than the other. These are evidently the two missing segments of the thorax, and instead of being in the process of being incorporated in the pygidium, they are in fact about to be cast off from it to become free thoracic segments. In other words, the thorax grows through the degeneration [137] of the pygidium. That the thorax grows at actual expense to the pygidium is shown by the proportions of this specimen. In an adult of this species the pygidium, thorax, and cephalon are to each other as 9:11:13. In the young specimen they are as 10:6:12, the pygidium being longer in proportion both to the thorax and to the cephalon than it would be in the adult.
This conception of the breaking down of the pygidium to form the thorax will be very helpful in explaining many things which have hitherto seemed anomalous. For instance, it indicates that the Agnostidæ, whose subequal shields in early stages have been a puzzle, are really primitive forms whose pygidia do not degenerate; likewise the Eodiscidæ, which, however, show within the family a tendency to free some of the segments. The annelidan Mesonacidæ may not be so primitive after all, and their specialized cephala may be more truly indicative of their status than has previously been supposed.
The facts of ontogeny of trilobites with both small and large pygidia do show that there is a reduction of the relative size of the caudal shield during the growth-stages, and therefore that the large pygidium in the protaspis is probably primitive. The same study also shows that the large pygidium is made up of "coalesced segments" only to the extent that they are potentially free, and not in the sense of fused segments.
That the narrow type of axial lobe is more primitive than the wide one has already been demonstrated by the ontogeny of various species, and space need not be taken here to discuss the question. Most Cambrian trilobites have narrow axial lobes even in the adult so that their development does not bring this out very strikingly, though it can be seen in Sao, Ptychoparia, etc., but in Ordovician trilobites such as Triarthrus and especially Isotelus, it is a conspicuous feature.
PRESENCE OR ABSENCE OF A "BRIM."
That the extension of the glabella to the front of the cephalon is a primitive feature is well shown by the development of Sao (Barrande, 1852, pl. 7), Ptychoparia (Beecher, 1895 C, pl. 8), and Paradoxides (Raymond, Bull. Mus. Comp. Zool., vol. 57, 1914), although in the last genus the protaspis has a very narrow brim, the larva during the stages of introduction of new segments a fairly wide one, and most adults a narrow one.
The brim of Sao seems to be formed partly by new growth and partly at the expense of the frontal lobe, for that lobe is proportionately shorter in the adult than in the protaspis. In Cryptolithus and probably in Harpes, Harpides, etc., the brim is quite obviously new growth and has nothing to do with the vital organs. Its presence or absence may not have any great significance, but when the glabella extends to the frontal margin, it certainly suggests a more anterior position of certain organs. In Sao, the only trilobite in which anything is known of the position of the hypostoma in the young, the posterior end is considerably further forward in a specimen a. 5 mm. long than in one 4 mm. long, thus indicating a backward movement of the mouth during growth, comparable to the backward movement of the eyes.
The very smallest specimens of Sao show a simple, unsegmented axial lobe, and the same simplicity has been noted in the young of other genera. Beecher considered this as [138] due to imperfect preservation of the exceedingly small shells, which practically always occur as moulds or casts in soft shale. There is, however, a very general increase in the strength of glabellar segmentation in the early part of the ontogeny of all trilobites whose life history is known, and in some genera, like the Agnostidæ, there is no question of the comparatively late acquisition of glabellar furrows. Even in Paradoxides, the furrows appear late in the ontogeny.
If absence of eyes on the dorsal surface be primitive, as Beecher has shown, and if the large pygidium, narrow axial lobe, and long unsegmented glabella be primitive, then the known protaspis of the Mesonacidæ and Paradoxidæ is not primitive, that of the Olenidæ is very primitive, and that of the Agnostidæ is primitive except that in one group the axial lobe, when it appears, is rather wide, and in the other a brim is present.
Subsequent development from the simple unsegmented protaspis would appear to show, first, an adaptation to swimming by the use of the pygidium; next, the invagination of the appendifers as shown in the segmentation of the axial lobe indicates the functioning of the appendages as swimming legs; then with the introduction of thoracic segments the assumption of a bottom-crawling habit is indicated. Some trilobites were fully adapted for bottom life, and the pygidium became reduced to a mere vestige in the production of a worm-like body. Other trilobites retained their swimming habits, coupled with the crawling mode of life, and kept or even increased (Isotelus) the large pygidium.
The Simplest Trilobite.
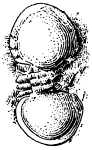
Fig. 35.—A specimen of Weymouthia nobilis (Ford), collected by Mr. Thomas H. Clark at North Weymouth, Mass. Note the broad smooth shields of this Lower Cambrian eodiscid. × 6.
In the discussion above I have placed great emphasis on the large size of the primitive pygidium, because, although there is nothing new in the idea, its significance seems to have been overlooked.
If the large pygidium is primitive, then multisegmentation in trilobites can not be primitive but is the result of adaptation to a crawling life. It is annelid-like, but is not in itself to be relied upon as showing relationship to the Chætopoda. Simple trilobites with few segments, like the Agnostidæ, Eodiscidæ etc., were, therefore, properly placed by Beecher at [139] the base of his classification, and there is now less chance than ever that they can be called degenerate animals.
From the phylogeny of certain groups, such as the Asaphidæ, it is learned that the geologically older members of the family have more strongly segmented anterior and posterior shields than the later ones. That there has been a "smoothing out" is demonstrated by a study of the ontogeny of the later forms. From such examples it has come to be thought that all smooth trilobites are specialized and occupy a terminal position in their genealogical line. This has caused some wonder that smooth agnostids like Phalacroma bibullatum and P. nudum should be found in strata so old as the Middle Cambrian, and was a source of great perplexity to me in the case of Weymouthia (Ottawa Nat., vol. 27, 1913) (fig. 35). This is a smooth member of the Eodiscidæ, and, in fact, one of the simplest trilobites known, for while it has three thoracic segments, it shows almost no trace of dorsal furrows or segmentation on cephalon or pygidium, and, of course, no eyes. Following the general rule, I took this to be a smooth-out eodiscid, and was surprised that it should come from the Lower Cambrian, where it is associated with Elliptocephala at Troy, New York, and with Callavia at North Weymouth, Massachusetts, and where it has lately been found by Kiær associated with Holmia and Kjerulfia at Tømten, Norway. It now appears it is really in its proper zone, and instead of being the most specialized, is the simplest of the Eodiscidæ.
What appears to be a still simpler trilobite is the form described by Walcott as Naraoia.
(Text fig. 36.)
Illustrated: Walcott, Smithson. Misc. Coll., vol. 57, 1912, p. 175, pl. 28, figs. 3, 4.—Cleland, Geology, Physical and Historical, New York, 1916, p. 412, fig. 382 F (somewhat restored).
This very imperfectly known form is referred by Walcott to the Notostraca on what appear to be wholly inadequate grounds, and while I do not insist on my interpretation, I can not refrain from calling attention to the fact that it can be explained as the most primitive of all trilobites. It consists of two subequal shields, the anterior of which shows slight, and the posterior considerable evidence of segmentation. It has no eyes, no glabella, and no thorax, and is directly comparable to a very young Phalacroma bibullatum (see Barrande 1852, pl. 49, figs. a, b). Walcott states that there is nothing to show how many segments there are in the cephalic shield, but that on one specimen fourteen were faintly indicated on the abdominal covering. The appendages are imperfectly unknown, as no specimen showing the ventral side has yet been described. The possible presence of antennas and three other appendages belonging to the cephalic shield is mentioned, and there are tips of fourteen legs projecting from beneath the side of one specimen. As figured, some of the appendages have the form of exopodites, others of endopodites, indicating that they were biramous.
Naraoia is, so far as now known, possessed of no characteristics which would prevent its reference to the Trilobita, while the presence of a large abdominal as well as a cephalic shield would make it difficult to place in even so highly variable a group as the Branchiopoda. On the other hand, its only exceptional feature as a trilobite is the lack of thorax, and all study of the ontogeny of the group has led us to expect just that sort of a trilobite to be found some day in the most ancient fossiliferous rocks. Naraoia can, I think, [140] be best explained as a trilobite which grew to the adult state without losing its protaspian form. It was found in the Middle Cambrian of British Columbia.
Even if Naraoia should eventually prove to possess characteristics which preclude the possibility of its being a primitive trilobite, it at least represents what I should expect a pre-Cambrian trilobite to look like. What the ancestry of the nektonic primitive trilobite may have been is not yet clear, but all the evidence from the morphology of cephalon, pygidium, and appendages indicates that it was a descendant of a swimming and not a crawling organism.
Since the above was written, the Museum of Comparative Zoology has purchased a specimen of this species obtained from the original locality. The shields are subequal, the posterior one slightly the larger, and the axial lobes are definitely outlined on both. The glabella is about one third the total width, nearly parallel-sided, somewhat pointed at the front. There are no traces of glabellar furrows. The axial lobe of the pygidium is also about one third the total width, extends nearly to the posterior margin, and has a rounded posterior end. The measurements are as follows: Length, 33 mm.; length of cephalon, 16 mm., width, 15 mm.; length of glabella, 11.5 mm., width, 5.5 mm.; length of pygidium, 17 mm., width, 15 mm.; length of axial lobe, 14 mm., width, 5.5 mm.
The species is decidedly Agnostus-like in both cephalon and pygidium, and were it not so large, might be taken for the young of such a trilobite. The pointed glabella is comparable to the axial lobes of the so called pygidia of the young of Condylopyge rex and Peronopsis integer (Barrande, Syst. Sil., vol. 1, pl. 49).
The Ancestor of the Trilobites, and the Descent of the Arthropoda.
The "annelid" theory of the origin of the Crustacea and therefore of the trilobites, originating with Hatschek (1877) and so ably championed by Bernard (1892), has now been a fundamental working hypothesis for some years, and has had a profound influence in shaping thought about trilobites. This hypothesis has, however, its weak points, the principal one being its total inhibition of the workings of that great talisman of the palæontologist, the law of recapitulation. Its acceptance has forced the zoologist to look upon the nauplius as a specially adapted larva, and has caused more than one forced explanation of the protaspis of the trilobite. When so keen a student as Calman says that the nauplius must point in some way to the ancestor of the Crustacea (1909, p. 26), it is time to reëxamine some of the fundamentals. This has been done in the preceding pages and evidence adduced to show that the primitive features of a trilobite indicate a swimming animal, and that the adaptations are those which enabled it to assume a crawling mode of existence. It has also been pointed out that in Naraoia there is preserved down to Middle Cambrian times an animal like that to which ontogeny points as a possible ancestor of the trilobites. Naraoia is not the simplest conceivable animal of its own type, however, for it has built up a pygidium of fourteen or fifteen somites. One would expect to find in Proterozoic sediments remains of similar animals with pygidia composed of only one or two somites, with five pairs of appendages on the cephalon, one or two pairs on the pygidium, a ventral mouth, and a short hypostoma. Anything simpler than this could not, in my opinion, be classed as a trilobite.
What the ancestor of this animal was is mere surmise. It probably had no test, and it may be noted in this connection that Naraoia had a very thin shell, as shown by its [141] state of preservation, and was in that respect intermediate between the trilobite and the theoretical ancestor. Every analysis of the cephalon of the trilobite shows that it is made up of several segments, certainly five, probably six, possibly seven. Every study of the trilobite, whether of adult, young, or protaspis, indicates the primitiveness of the lateral extensions or pleural lobes. The same studies indicate as clearly the location of the vital organs along the median lobe. These suggestions all point to a soft-bodied, depressed animal composed of few segments, probably with simple marginal eyes, a mouth beneath the anterior margin, tactile organs at one or both ends, with an oval shape, and a straight narrow gut running from anterior mouth to terminal anus. The broad flat shape gives great buoyancy and is frequently developed in the plankton. Inherited by the trilobites, it proved of great use to the swimmers among them.
The known animal which most nearly approaches the form which I should expect the remote ancestor of the trilobites to have had is Amiskwia sagittiformis Walcott (Smithson. Misc. Coll., vol. 57, 1911, p. 112, pl. 22, figs. 3, 4). This "worm" from the Middle Cambrian is similar in outline to the recent Spadella, and is referred by Walcott to the Chætognatha. It has a pair of lateral expansions and a flattened caudal fin, a narrow median alimentary canal, and a pair of rather long simple tentacles. With the exception of a thin septum back of the head, no traces of segmentation are shown.
Some time in the late pre-Cambrian, the pre-trilobite, which probably swam by rhythmic undulations of the body, began to come into occasional contact with a substratum, and two things happened: symmetrically placed, i. e., paired, appendages began to develop on the contact surface, and a test on the dorsal side. The first use of the appendages may have been in pushing food forward to the mouth, and for the greater convenience in catching such material, a fold in front of the mouth may have elongated to form the prototype of the hypostoma. At this time the substratum may not have been the ocean bottom at all, but the animals, still free swimmers, may have alighted at feeding time on floating algæ from the surface of which they collected their food. While the dorsal test was originally jointed at every segment, the undulatory mode of swimming seems to have given way to the method of sculling by means of the posterior end only, or by the use of the appendages, and the anterior segments early became fused together.
The result of the hardening of the dorsal test was of course to reduce to that extent the area available for respiration, and this function was now transferred in part to the limbs, which bifurcated, one branch continuing the food-gathering process and the other becoming a gill. The next step may have been the "discovery" of the ocean bottom and the tapping of an hitherto unexploited supply of food. Upon this, there set in those adaptations to a crawling mode of existence which are so well shown in the trilobite. The crawling legs became lengthened and took on a hardened test, the hypostoma was greatly elongated, pushing the mouth backward, and new segments were added to produce a long worm-like form which could adapt itself to the inequalities of the bottom. That the test of the appendages became hardened later than that of the body is shown by the specimens of Neolenus, in which the dorsal shell as preserved in the shale is thick and solid, while the test of the appendages is a mere film.
The late Proterozoic or very earliest Cambrian was probably the time of the great splitting up into groups. The first development seems to have been among the trilobites themselves, the Hypoparia giving rise to two groups with compound eyes, first the Opisthoparia and later the Proparia. About this same time the Copepoda may have split off [142] from the Hypoparia, continuing in the pelagic habitat. At first, most of the trilobites seem to have led a crawling existence, but about Middle Cambrian time they began to go back partially to the ancestral swimming habits, and retained some of the trunk segments to form a larger pygidium. The functional importance of the pygidium explains why it can not be used successfully in making major divisions in classification. Nearly related trilobites may be adapted to diverse methods of life.
EVOLUTION WITHIN THE CRUSTACEA.
The question naturally arises as to whether the higher Crustacea were derived from some one trilobite, or whether the different groups have been developed independently from different stocks. The opinion that all other crustaceans could have been derived from an Apus-like form has been rather generally held in recent years, but Carpenter (1903, p. 334) has shown that the leptostracan, Nebalia, is really a more primitive animal than Apus. He has pointed out that in Leptostraca the thorax bears eight pairs of simple limbs with lamelliform exopodites and segmented endopodites, while the abdomen of eight segments has six pairs of pleopods and a pair of furcal processes, so that only one segment is limbless. Contrasted with this are the crowded and complicated limbs of the anterior part of the trunk of Apus, and the appendage-less condition of the hinder portion. Further, a comparison between the appendages of the head of Nebalia and those of Apus shows that the former are the more primitive. The antennules of Nebalia are elongate, those of Apus greatly reduced; the mandible of Nebalia has a long endopodite, and Carpenter points out that from it either the malacostracan mandible with a reduced endopodite or the branchiopodan mandible with none could be derived, but that the former could not have arisen from the latter. The maxillæ of Apus are also much the more specialized and reduced.
Nebalia being in all else more primitive than Apus, it follows that the numerous abdominal segments of the latter may well have arisen by the multiplication of an originally moderate number, and the last trace of primitiveness disappears.
It is now possible to add to the results obtained from comparative morphology the testimony of palæontology, already outlined above, and since the two are in agreement, it must be admitted that the modern Branchiopoda are really highly specialized.
As has already been pointed out, Hymenocaris, the leptostracan of the Middle Cambrian, has very much the same sort of appendages as the Branchiopoda of the same age, both being of the trilobite type. Which is the more primitive, and was one derived from the other?
The Branchiopoda were much more abundant and much more highly diversified in Cambrian times than were the Leptostraca, and, therefore, are probably older. Some of the Cambrian branchiopods were without a carapace, and some were sessile-eyed. These were more trilobite-like than Hymenocaris. Many of the Cambrian branchiopods had developed a bivalved carapace, though not so large a one as that of the primitive Leptostraca. The present indications are, therefore, that the Branchiopoda are really older than the Leptostraca, and also that the latter were derived from them. It seems very generally agreed that the Malacostraca are descended from the Leptostraca, and the fossils of the Pennsylvanian supply a number of links in the chain of descent. Thus, Pygocephalus cooperi, with its brood pouches, is believed by Calman (1909, p. 181) to stand at the base of the Peracaridan series of orders, and Uronectes, Palæocaris, and the like are Palæozoic representatives of the Syncarida. Others of the Pennsylvanian species appear to tend in the direction of the Stomatopoda, [143] whose true representatives have been found in the Jurassic. The Isopoda seem to be the only group of Malacostraca not readily connected up with the Leptostraca. Their depressed form, their sessile-eyes, and their antiquity all combine to indicate a separate origin for the group, and it has already been pointed out how readily they can be derived directly from the trilobite.
While the Copepoda seem to have been derived directly from the Hypoparia, the remainder of the Crustacea apparently branched off after the compound eyes became fully developed, unless, as seems entirely possible, compound eyes have been developed independently in various groups. Most Crustacea were derived from crawling trilobites (Lower Cambrian or pre-Cambrian Opisthoparia), for they lost the large pygidium, and also the major part of the pleural lobes. In all Crustacea, too, other than the Copepoda and Ostracoda, there is a tendency to lose the exopodites of the antennæ.
These modifications, which produced a considerable difference in the general appearance of the animal, are easily understood. As has been shown in previous pages, the trilobites themselves exhibit the degenerative effect on the anterior appendages of the backward movement of the mouth, and the transformation of a biramous appendage with an endobase into a uniramous antenna is a simple result of such a process. The feeding habits of the trilobites were peculiar and specialized, and it is natural that some members of the group should have broken away from them. In any progressive mode of browsing the hypostoma was a hindrance, so was soon gotten rid of, and the endobases not grouped around the mouth likewise became functionless. The chief factor in the development of the higher Crustacea seems to have been the pinching claw, by means of which food could be conveyed to the mouth. It had the same place in crustacean development that the opposable thumb is believed to have had in that of man.
An intermediate stage between the Trilobita and the higher Crustacea is at last exhibited to us by the wonderful, but unfortunately rather specialized Marrella, already described. It retains the hypostoma and the undifferentiated biramous appendages of the trilobite, but has uniramous antennæ, there are no endobases on the coxopodites of the thoracic appendages, the pygidium is reduced to a single segment, and the lateral lobes of the thorax are also much reduced. Marrella is far from being the simplest of its group, but is the only example which survived even down to Middle Cambrian times of what was probably once an important series of species transitional between the trilobites and the higher Crustacea.
In this theory of the origin of the Crustacea from the Trilobita, the nauplius becomes explicable and points very definitely to the ancestor. According to Calman (1909, p. 23):
The typical nauplius has an oval unsegmented body and three pairs of limbs, corresponding to the antennules, antennas, and mandibles of the adult. The antennules are uniramous, the others biramous, and all three pairs are used in swimming. The antennæ may have a spiniform or hooked masticatory process at the base, and share with the mandibles which have a similar process, the function of seizing and masticating the food. The mouth is overhung by a large labrum or upper lip and the integument of the dorsal surface of the body forms a more or less definite dorsal shield. The paired eyes are as yet wanting, but the median eye is large and conspicuous.
The large labrum or hypostoma, the biramous character of the appendages, especially of the antennæ, the functional gnathobases on the second and third appendages, and the oval unsegmented shield are all characteristics of the trilobites, and it is interesting to note that all nauplii have the free-swimming habit.
The effect of inheritance and modification through millions of generations is also shown in the nauplius, but rather less than would be expected. The most important modification [144] is the temporary suppression of the posterior pairs of appendages of the head, so that they are generally developed later than the thoracic limbs. The median or nauplius eye has not yet been found in trilobites, and if it is, as it appears to be, a specialized eye, it has probably arisen since the later Crustacea passed the trilobite stage in their phylogeny.
The oldest Crustacea, other than trilobites, so far known are the Branchiopoda and Phyllocarida described by Walcott and discussed above. It is important to note that while the former have already achieved such modified characteristics that they have been referred to modern orders, they retain the trilobite-like limbs and some of them still have well developed pleural lobes.
Calman (1909, p. 101) says of the Copepoda:
On the hypothesis that the nauplius represents the ancestral type of the Crustacea, the Eucopepoda would be regarded as the most primitive existing members of the class, retaining as they do, naupliar characters in the form of the first three pairs of appendages and in the absence of paired eyes and of a shell-fold. As already indicated, however, it is much more probable that they are to be regarded as a specialized and in some respects degenerate group which, while retaining, in some cases, a very primitive structure of the cephalic appendages, has diverged from the ancestral stock in the reduction of the number of somites, the loss of the paired eyes and the shell-fold, and the simplified form of the trunk-limbs.
If the Eucopepoda be viewed in the light of the theory of descent here suggested, it is at once seen that while they are modified and specialized, they more nearly approximate the hypothetical ancestor than any other living Crustacea. Compound eyes are absent, and it can not be proved that they were ever present, although Grobben is said to have observed rudiments of them in the development of Calanus. The "simplified limbs" are the simple limbs of the trilobite, somewhat modified. The absence of the shell-fold and carapace is certainly a primitive characteristic. Add to this the direct development of the small number of segments, and the infolded pleural lobes, and it must be admitted that the group presents more trilobite-like characteristics than any other. It seems very likely that the primitive features were retained because of the pelagic habitat of a large part of the group.
Ruedemann (Proc. Nat. Acad. Sci., vol. 4, 1918, p. 382, pl.) has recently outlined a possible method of derivation of the acorn barnacles from the phyllocarids. Starting from a recent Balanus with rostrum and carina separated by two pairs of lateralia, he traces back through Calophragmus with three pairs of lateralia to Protobalanus of the Devonian with five pairs. Still older is the newly discovered Eobalanus of the upper Ordovician, which also has five pairs of lateralia but the middle pair is reversed, so that when the lateralia of each side are fitted together, they form a pair of shields like those of Rhinocaris, separated by the rostrum and carina, which are supposed to be homologous with the rostrum and dorsal plate of the Phyllocarida. Ruedemann suggests that the ancestral phyllocarid attached itself by the head, dorsal side downward, and the lateralia were developed from the two valves of the carapace during its upward migration, to protect the ventral side exposed in the new position.
This theory is very ingenious, but has not been fully published at the time of writing, and it seems very doubtful if it can be sustained.
The salient points in the preceding discussion should be disentangled from their setting and put forward in a brief summary.
It is argued that the ancestral arthropod was a short and wide pelagic animal of few segments, which so far changed its habits as to settle upon a substratum. As a result of change in feeding habits, appendages were developed, and, due perhaps to physiological change induced by changed food, a shell was secreted on the dorsal surface, covering the whole body. Such a shell need not have been segmented, and, in fact, the stiffer the shell, the more reason for development of the appendages. Activity as a swimming and crawling animal tended to break up the dorsal test into segments corresponding to those of the soft parts, and, by adaptation, a floating animal became a crawling one, with consequent change from a form like that of Naraoia to one like Pædeumias. (See figs. 36-40.) A continuation of this line of development by breaking up and loss of the dorsal test led through forms similar to Marrella to the Branchiopoda of the Cambrian, in which not only is there great reduction in the test, but also loss of appendages. The origin of the carapace is still obscure, but Bernard (1892, p. 214, fig. 48) has already pointed out that some trilobites, Acidaspidæ particularly, have backward projecting spines on the posterior margin of the cephalon, which suggest the possibility of the production of such a shield, and in Marrella such spines are so extravagantly developed as almost to confirm the probability of such [146] origin. In this line of development two pairs of tactile antennæ were produced, while the anomomeristic character of the trilobite was retained. From similar opisthoparian ancestors there were, however, derived primitive Malacostraca retaining biramous antennæ, but with a carapace and reduced pleural lobes and pygidium. From this offshoot were probably derived the Ostracoda, the Cirripedia, and the various orders of the Malacostraca, with the possible exception of the Isopoda. I have suggested independent origins of the Copepoda and Isopoda, but realize the weighty arguments which can be adduced against such an interpretation.
![]() |
![]() |
Fig. 36.—Naraoia compacta Walcott. An outline of the test, after Walcott. Natural size. |
Fig. 37.—Pagetia clytia Walcott. An eodiscid with compound eyes. After Walcott. × 5. |
It is customary to speak of the Crustacea and Trilobita as having had a common ancestry, rather than the former being in direct line of descent from the latter, but when it can be shown that the higher Crustacea are all derivable from the Trilobita, and that they possess no characteristics which need have been inherited from any other source than that group, it seems needless to postulate the evolution of the same organs along two lines of development.
I can not go into the question of which are more primitive, sessile or stalked eyes, but considering the various types found among the trilobites, one can but feel that the stalked eyes are not the most simple. While no trilobite had movable stalked eyes, it is possible to homologize free cheeks with such structures. They always bear the visual surface, and, in certain trilobites (Cyclopyge), the entire cheek is broken up into lenses. Since a free cheek is a separate entity, it is conceivable that it might lie modified into a movable organ.
It has been pointed out above that the Limulava (Sidneyia, Amiella, Emeraldella) have certain characteristics in common with the trilobites on the one hand and the Eurypterida on the other. These relationships have been emphasized by Walcott, who derives the Eurypterida through the Limulava and the Aglaspina from the Trilobita. The Limulava may be derived from the Trilobita, but indicate a line somewhat different from that of the remainder of the Crustacea. In this line the second cephalic appendages do not become antennæ. and the axial lobe seems to broaden out, so that the pleural lobes become an integral part of the body. As in the modern Crustacea, the pygidium is reduced to the anal plate, and this grows out into a spine-like telson.
From the Limulava to the Eurypterida is a long leap, and before it can be made without danger, many intermediate steps must be placed in position. The direct ancestor of the Eurypterida is certainly not to be seen in the highly specialized Sidneyia, and probably not in Emeraldella, but it might be sought in a related form with a few more segments. The few species now known do suggest the beginning of a grouping of appendages about the mouth, a suppression of appendages on the abdomen, and a development of gills on the thorax only. Further than that the route is uncertain.
Clarke and Ruedemann, whose recent extensive studies give their opinion much weight, seem fully convinced that the Merostomata could not have been derived from the Trilobita, but are rather inclined to agree with Bernard that the arachnids and the crustaceans were derived independently from similar chætopod annelids (1912, p. 148).
The greater part of their work was, however, finished before 1910, and although they refer to Walcott's description of the Limulava (1911), they did not have the advantage of studying the wonderful series of Crustacea described by him in 1912. While the evidence [147] is far from clear, it would appear that the discovery of animals with the form of Limiting and the eurypterids and the appendages of trilobites means something more than descent from similar ancestors. Biramous limbs of the type found in the trilobites would probably not be evolved independently on two lines, even if the ancestral stocks were of the same blood.
The Aglaspidæ, as represented by Molaria and Habelia in the Middle Cambrian, are quite obvious closely related to the trilobites easily derived from them, and retain numerous of their characteristics. That they are not trilobites is, however, shown by the presence of two pairs of antennæ, the absence of facial sutures, and the possession of a spine-like telson.
The Aglaspidæ have always been placed in the Merostomata, and nearer the Limulidæ than the Eurypterida. The discovery of appendages does not at all tend to strengthen that view, but indicates rather that they are true Crustacea which have not given rise to any group now known. The exterior form is, however, Limulus-like, and since it is known from ontogeny that the ancestor of that genus was an animal with free body segments, there is still a temptation to try to see in the Aglaspidæ the progenitors of the limulids.
The oldest known Limulus-like animal other than the Aglaspidæ is Neolimulus falcatus Woodward (Geol. Mag., dec. 1, vol. 5, 1868, p. 1, pl. 1, fig. 1). The structure of the head of this animal is typically limuloid, with simple and compound eyes and even the ophthalmic ridges. Yet, curiously enough, it shows what in a trilobite would be considered the posterior half of the facial suture, running from the eye to the genal angle. The body is composed of eight free segments with the posterior end missing. Belinurus, from the Mississippian and Pennsylvanian, has a sort of pygidium, the posterior three segments being fused together, and Prestwichia of the Pennsylvanian has all the segments of the abdomen fused together. So far as form goes, a very good series of stages can be selected, from the Aglaspidæ of the Cambrian through Neolimulus to the Belinuridæ of the late Palæozoic and the Limulidæ of the Mesozoic to recent. Without much more knowledge of the appendages than is now available, it would be quite impossible to defend such a line. It is, however, suggestive.
The trilobites were such abundant and highly variable animals, adapting themselves to various methods of life in the sea, that it appears highly probably that some of them may have become adapted to life on the land. The ancestors of the Chilopoda, Diplopoda, and Insecta appear to have been air-breathing animals as early as the Cambrian, or at latest, the Ordovician. Since absolutely nothing is yet known of the land or even of the fresh-water life of those periods, nothing can now be proved.
In discussing the relationship of the trilobites to the various tracheate animals, I have pointed out such palæontologic evidence as I have been able to gather. Studies in the field of comparative morphology do not fall within my province. I only hope to have made the structure of the trilobite a little more accessible to the student of phylogenies.
In order to put into graphic and concise form the suggestions made above, it is necessary to define and give names to some of the groups outlined. The hypothetical ancestor [148] need not be included in the classification and for reasons of convenience may be referred to merely as the Protostracean.
The group of free-swimming trilobites without thoracic segments was probably a large one, and within it there were doubtless considerable variations and numerous adaptations. While the only known animal which could possibly be referred to this group, Naraoia, is blind, it is entirely possible that other species had eyes, and that the cephala and pygidia were variously modified. For this reason and because of the lack of all thoracic segments, it seems better to erect a new order rather than merely a family for the group, and Nektaspia (swimming shields) may be suggested. The only known family is Naraoidæ Walcott, which must be redefined.
Marrella and Habelia are types of Crustacea which can neither be placed in the Trilobita nor in any of the established subclasses of the Eucrustacea. They represent a transitional group, the members of which are, so far as known, adapted to the crawling mode of life, though it may prove that there are also swimmers which can be classified with them. To this subclass the name Haplopoda may be applied, the feet being simple.
The two known families, Marrellidæ Walcott and Aglaspidæ Clarke, belong to different orders, the second having already the name Aglaspina Walcott. The name Marrellina may therefore be used for the other.
For Sidneyia, Walcott proposed the new subordinal name Limulava, placing it under the Eurypterida. While Sidneyia, Emeraldella, and Amiella may belong to the group that gave rise to the Eurypterida, they are themselves Crustacea, and a place must be found for them in that group. The possession of only one pair of antennæ prevents their reception by the Haplopoda, and allies them to the Trilobita, but the modifications of the trunk and its appendages keep them out of that subclass, and a new one has to be erected for them. This may be known as the Xenopoda, in allusion to the strange appendages of Sidneyia.
Synopsis.
Class Crustacea.
Subclass Trilobita Walch.
Crustacea with one pair of uniramous antennæ, and possessing facial sutures.
Order Nektaspia nov.
Trilobita without thoracic segments. Cephala and pygidia simple.
Family Naraoidæ Walcott.
Cephalon and pygidium large, both shields nearly smooth. Eyes absent. A single species: Naraoia compacta Walcott, Middle Cambrian, British Columbia.
Subclass Haplopoda nov.
Crustacea with trilobate form, two pairs of uniramous antennæ, no facial sutures, sessile compound eyes present or absent, pygidium and pleural lobes generally reduced, large labrum present, appendages of the trunk biramous.
Order Marrellina nov.
Form trilobite-like, pleural lobes reduced, endobases absent from coxopodites of body, pygidium a small plate.
Family Marrellidæ Walcott.
Cephalon with long genal and nuchal spines. Eyes marginal. A single species: Marrella splendens Walcott, Middle Cambrian, British Columbia.
Order Aglaspina Walcott.
Body trilobite-like, with few thoracic segments, and a spine-like telson. Appendages biramous.
Family Aglaspidæ Clarke.
Cephalon trilobate, with or without compound eyes, seven or eight segments in the thorax.
Genus Aglaspis Hall.
Compound eyes present, seven segments in thorax. Upper Cambrian, Wisconsin.
Genus Molaria Walcott.
Compound eyes absent, eight segments in thorax. Middle Cambrian, British Columbia.
Genus Habelia Walcott.
Compound eyes absent. Not yet fully described. Middle Cambrian, British Columbia.
Subclass Xenopoda nov.
Crustacea with more or less eurypterid-like form, one pair of uniramous antennæ, biramous appendages on anterior part of trunk, modified endopodites on cephalon.
Order Limulava Walcott.
Cephalon with lateral or marginal eyes and large epistoma. Body with eleven free segments and a telson. Cephalic appendages grouped about the mouth.
Family Sidneyidæ Walcott.
Trunk probably with exopodites only, and without appendages on the last two segments. Telson with a pair of lateral swimmerets.
Genus Sidneyia Walcott.
Third cephalic appendage a large compound claw. Gnathobases forming strong jaws. Middle Cambrian, British Columbia.
Genus Amiella Walcott.
Middle Cambrian, British Columbia.
Family Emeraldellidæ nov.
Trunk with biramous appendages in anterior part, and appendages on all segments except possibly the spine-like telson.
Genus Emeraldella Walcott.
Cephalic appendages simple spiniferous endopodites. Eyes unknown. Middle Cambrian, British Columbia.
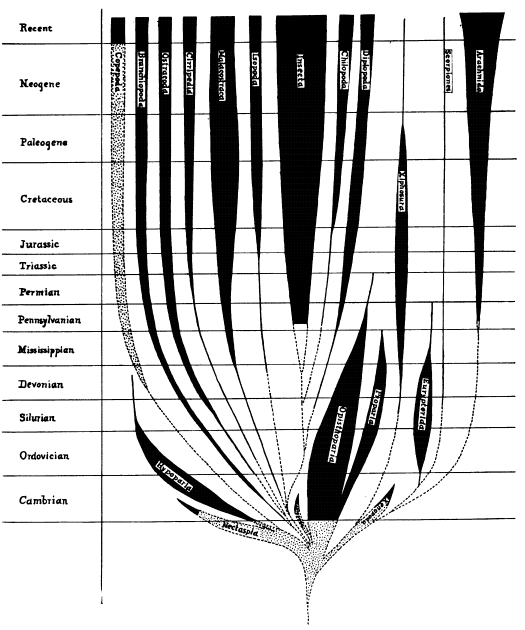
Click on image to view larger sized
Fig. 41.—A diagram showing possible lines of descent of the other Arthropoda from the Trilobita. The three recognized orders of the latter are shown separately. The known geological range is indicated in solid black, the hypothetical range and connections stippled. The short branch beside the Opisthoparia represents the range of the Haplopoda. The term Arachnida is used for all arachnids other than Merostomata, merely as a convenient inclusive name for the groups not especially studied.
Final Summary.
It is generally believed that the Arthropoda constitute a natural, monophyletic group. The data assembled in the preceding pages indicate that the other Arthropoda were derived directly or indirectly from the Trilobita because:
(1) the trilobites are the oldest known arthropods;
(2) the trilobites of all formations show great variation in the number of trunk segments, but with a tendency for the number to become fixed in each genus;
(3) the trilobites have a constant number of segments in the head;
(4) the position of the mouth is variable, so that either the Crustacea or the Arachnida could be derived from the trilobites;
(5) the trilobite type of appendage is found, in vestigial form at least, throughout the Arthropoda;
(6) the appendages of all other Arthropoda are of forms which could have been derived from those of trilobites;
(7) the appendages of trilobites are the simplest known among the Arthropoda;
(8) the trilobites show practically all known kinds of sessile arthropodan eyes, simple, compound, and aggregate;
(9) the apparent specializations of trilobites, large pleural lobes and pygidia, are primitive, and both suffer reduction within the group.
The ancestor of the trilobite is believed to have been a soft-bodied, free-swimming, flat, blind or nearly blind animal of few segments, because:
(a) the form of both adult and embryo is of a type more adapted for floating than crawling;
(b) the large pygidium is shown by ontogeny to be primitive, and the elongate worm-like form secondary;
(c) the history of the trilobites shows a considerable increase in the average number of segments in successive periods from the Cambrian to the Permian;
(d) the simplest trilobites are nearly or quite blind.
PART IV.
DESCRIPTION OF THE APPENDAGES OF INDIVIDUAL SPECIMENS.
In order to make easily available the evidence on which the present knowledge of the appendages of Triarthrus and Cryptolithus rests, it has seemed wise to publish brief descriptions and photographic figures of some of the better specimens preserved in the Yale University Museum. These specimens are pyritic replacements, and while they do not as yet show any signs of decomposition, it should be realized that it is only a matter of time when either they will be self-destroyed through oxidation, or else embedded for safe keeping in such a fashion that they will not be readily available for study. It is therefore essential to keep a photographic record of the more important individuals.
Specimen No. 220 (pl. 3, fig. 2).
Illustrated: Amer. Geol., vol. 15, 1895, pl. 4 (drawing); Amer. Jour. Sci., vol. 13, 1902, pl. 3 (photograph).
This is one of the largest specimens showing appendages, and is developed from the ventral side. It shows some appendages on all parts of the body, but its special features are the exhibition of the shafts on the proximal ends of the antennules, the rather well preserved appendages of the cephalon and anterior part of the thorax, and the preservation of the anal opening. In the drawing in the American Geologist, the right and left sides are reversed as in a mirror, a point which should be borne in mind when comparing that figure with a photograph or description.
The shaft of the left antennule is best preserved and is short, cylindrical, somewhat enlarged and ball-shaped at the proximal end. It is 1.5 mm. long. The posterior part of the hypostoma is present, but crushed, and the metastoma is not visible, the pieces so indicated in Beecher's figure being the rim of the hypostoma. Back of the hypostoma may be seen four (not three as in Beecher's figure) pairs of gnathites, the first three pairs broad and greatly overlapping, the fourth pair more slender, but poorly preserved. The inner edges of the gnathites on the right side are distinctly nodulose, and roughened for mastication.
The outer ends of one endopodite and three exopodites project beyond the margin on the right side. The dactylopodite of the endopodite is especially well preserved. It is cylindrical, the end rounded but not enlarged or pointed, and bears three small sharp spines, all in a horizontal plane, one anterior, one central, and one posterior. The outer ends of the exopodites show about ten segments each (in 2.5 mm.) beyond the margin of the test, and from three to five setæ attached to the posterior side of each segment. These hairs are attached in a groove, well shown in this specimen. On the anterior margin of the exopodite there is a minute spine at each joint.
Measurements: Length, 38 mm.; width at back of cephalon, 19 mm.
Specimen No. 210 (pl. 2, fig. 3).
Illustrated: Amer. Jour. Sci., vol. 46, 1893, p. 469, fig. 1 (head and right side); Amer. Geol., vol. 13, 1894, pl. 3, fig. 7 (same figure as the last); Amer. Jour. Sci., vol. 13, 1902, pl. 2, fig. 1 (photograph).
This individual supplied the main basis for Professor Beecher's first figure showing the appendages of the thorax, the head and appendages of the right side having been taken [153] from it, and the appendages of the left side from No. 206. Such of the endopodites as are well preserved show from three to four segments projecting beyond the test, and the dactylopodites have one or two terminal spines. The antennules are unusually well preserved and have about forty segments each in front of the cephalon, or an average of five to one millimeter.
Specimens 209 and 210 are on a slab about 7 × 5.5 inches, and with them are twelve other more or less well preserved individuals, all but one of which are smaller than these. Two of the fourteen are ventral side up on the slab, which means dorsal side up in the rock. Nine are oriented in one direction, two at exactly right angles to this, and three at an angle of 45 with the others. If the majority of the specimens are considered to be headed northward, then seven are so oriented, two northeast, one east, two south, one southwest, and one west.
Nine of the specimens show antennules. Five of these are specimens headed north, and in all of them the antennules are in or very near the normal position. The antennules of two, one headed east and the other west, are imperfectly preserved, but the parts remaining diverge much more than do the antennules of those in the normal position. The individual headed southwest has one antennule broken off, while the other is curved back so that its tip is directed northward. Another one, headed south, has the antennules in the normal position. These observations indicate that the specimens were oriented by currents of water, rather than in life attitudes, and that the distal portions of the antennules were relatively flexible.
Measurements: The specimen (No. 210) is 20 mm. long, 9.5 mm. wide at the back of the cephalon, and the antennules project 8 mm. in front of the head. The smallest specimen on the slab is 6.5 mm. long. A specimen 7.5 mm. long has antennules which project 2.5 mm. in front of the cephalon.
Specimen No. 201 (pl. 2, fig. 1; pl. 3, fig. 4).
Illustrated: Amer. Jour. Sci., vol. 46, 1893, p. 469, figs. 2, 3; Amer. Geol., vol. 13, 1894, pl. 3, figs. 8, 9.
An entire specimen 17 mm. long, exposed from the dorsal side. It shows only traces of the appendages of the head, but displays well those of the anterior part of the thorax, and a number of appendages emerge from under the abdominal shield. This specimen is of particular interest as it is the subject of the first of Professor Beecher's papers on appendages of trilobites. On the right side the pleura have been removed, so as to expose the appendages of the second, third, and fourth segments from above. The first two of the appendages on the right are best preserved, and these are the ones figured. They belong to the second and third segments. The endopodites of each are ahead of the exopodites, and the proximal portion of each exopodite overlies portions of the first two segments (second and third) of the corresponding endopodite. The coxopodites are not visible, but very nearly the full length of the first segment of the endopodite (the basipodite) is exposed. The first two visible segments (the first and second) extend just to the margin of the pleural lobe, while the other four extend beyond the dorsal cover. The segments decrease in length outward, but not regularly, the meropodite being generally longer than the ischiopodite or the carpopodite. The terminal segment (dactylopodite) is short and bears short sharp hair-like spines which articulate in sockets at the distal end. On this specimen the anterior limb on the right side shows one terminal spine, the second endopodite on that side has two, and two of the endopodites on the left-hand side preserve two each. The segments of the limbs [154] are nearly cylindrical, but the ischiopodites and meropodites of several of the endopodites show rather deep longitudinal grooves which appear to be rather the result of the shrinkage of the thin test than natural conformations.
The endopodites on the left-hand side have a number of short, sharp, movable, hair-like spines, and cup-shaped depressions which are the points of insertion of others. On the distal end of the carpopodite of the first thoracic segment there seems to have been a spine, whose place is now shown by a pit. This same endopodite shows, rather indistinctly, three pits in the groove of the carpopodite, and the propodite has two. On the endopodite of the second appendage on this side, both the carpopodite and propodite possess a fine hair-like articulated spine at the distal end, that of the propodite arising on the dorsal and that of the carpopodite on the posterior side. On the dorsal side of the carpopodite there are three pits for the articulation of spines, and on the propodite, one.
The exopodites belonging to the thoracic segments are of equal length with the endopodites, and while the proximal portion of each is stouter than that of the corresponding endopodite, the exopodites taper to a hair-like termination, while the endopodites remain fairly stout to the distal segment. Most of the setæ of the exopodites have been removed, so that each remains as a curving, many-segmented organ, transversely striated, with a continuous groove along the posterior side. The setæ appear to be set in this groove, one for each of the transverse ridges on the shaft.
A good deal of the test has been cut away on the left-hand side from the thorax and pygidium, and the appendages exposed from above. Enough of the dorsal shell has been cut away so that the anal opening is exposed, and directly behind the pygidium, on the median line, is a bilaterally symmetrical plate with serrated edges which appears to be the appendage of the anal segment. (See pl. 3, fig. 4.)
Measurements: The specimen is 17 mm. long, and 8 mm. in greatest width (at the back of the cephalon). From the median tubercle to the outer edge of the pleuron of the second thoracic segment the distance is 3.7 mm. From the point of articulation to the distal end of the spines on the dactylopodite of the second endopodite on the right-hand side is 4.3 mm. The basipodite of this appendage is 1.5 mm. long, the ischiopodite 1 mm. long, the meropodite 1.2 mm. long, the carpopodite 0.5 mm. long, the propodite 0.35 long, and the dactylopodite 0.15 mm. long. On the left-hand side the endopodite of the first segment projects 3 mm. beyond the pleuron, the second, 3.2 mm. At the back the appendages extend a maximum distance of 2.5 mm. behind the pygidium. The median spinose process of the anal segment extends 0.75 mm. behind the pygidium, and is 1.6 mm. in greatest width.
Specimen No. 204 (pl. 3, fig. 1; pl. 4, fig. 6; text fig. 42).
Illustrated: Amer. Jour. Sci., vol. 13, 1902, pl. 2, figs. 4, 5 (reproduced from photographs).
This specimen, which is developed from the dorsal surface, shows especially well nine appendages of the left side. The first represent the last segment of the cephalon; the remainder belong to the thorax. As is usual, the exopodites of these appendages overlie and curve behind the endopodites. All the exopodites have lost their setæ and the segments of the endopodites are flattened by crushing. The endopodites, while retaining only one or two of the movable spines, have the cup-like bases of from two to four on each of the visible segments, namely, the meropodite, carpopodite, propodite, and, in one case, the dactylopodite. These appendages, although really marvellous in preservation, are of such small size and react so badly to light that their study is very difficult, and Professor Beecher, who had [155] observed hundreds of specimens through all stages of the laborious process of cleaning the matrix from them, undoubtedly was much better equipped to interpret them than any other person.
The drawing is made on the assumption that the appendages are displaced and all moved uniformly outward so that the distal ends of the coxopodites emerge from under the pleural lobe, whereas these ends would normally be under the dorsal furrow, and the distal end of the ischiopodite should reach the margin of the pleural lobe. While it seems very remarkable that it should happen, that all the appendages should be so moved that they would lie symmetrically a few millimeters from their normal position, nevertheless it is found on measuring that they bear the same proportion to the length and width that the appendages of other specimens do, thus indicating that Professor Beecher's interpretation of them was correct. I am unable, however, to see the coxopodites which he has drawn as articulating with the two branches of the limb.
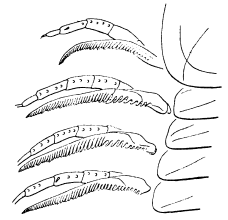
Fig. 42.—Triarthrus becki Green. Appendages of specimen 204. Inked in by Miss Wood from the original tracing. × 10.
This individual shows, better than any other, the connection of the exopodite with the endopodite. Even though the coxopodites are gone, the two branches of each appendage remain together, showing that the basipodite as well as the coxopodite is involved in the articulation with the exopodite. Just what the connection is can not be observed, but there seems to be a firm union between the upper surface of the basipodite and the lower side of the proximal end of the exopodite, as indicated diagrammatically in text figure 33.
Measurements: The specimen is 20 mm. long and 9 mm. wide at the back of the cephalon. From the tubercle on the middle of the first segment of the thorax to the tip of the corresponding appendage the distance is 8 mm. The entire length of the exopodite of the first thoracic segment is 4.6 mm. The exopodite of the appendage belonging to the seventh segment is only 3.5 mm. long. The pleural lobe is 2.5 mm. wide at the front of the thorax.
Specimen No. 205 (pl. 2, fig. 4).
Illustrated: Amer. Jour. Sci., vol. 13, 1902, pl. 5, figs. 2, 3 (photographs).
This is a small imperfect specimen, developed from the ventral side. It retains the best preserved metastoma in the collection, but was used by Professor Beecher especially to illustrate [156] the convergent ridges on the inside of the ventral membrane in the axial region of the thorax. These ridges are very low, and on each segment of the thorax there is a central one, outside of which is a pair which are convergent forward, making angles of 35 to 45 with the axis.
The metastoma is shaped much like the hypostoma of an Illænus. It is convex, nearly semicircular, with the straight side forward, and there is a continuous raised border around the curved sides and back. This border is separated from the central convex body by a deep linear depression.
The hypostoma is also rather well preserved and has a narrow, slightly elevated border at the sides and back.
Measurements: The incomplete specimen, from which only a very small portion of the length is missing, is 9 mm. long. The metastoma is 0.45 mm. long and 0.58 mm. wide.
Specimen No. 214 (pl. 1, fig. 2; pl. 3, fig. 6).
This is a large specimen, developed from the ventral side. It shows the antennules and some other appendages of the head, but derives its special interest from the excellent preservation of a few of the exopodites, which are turned back parallel to the axis of the body and lie within the axial lobe.
The shaft of the exopodite is made up of numerous short segments which at their anterior outer angles are produced into spines, and which also bear movable spines along the anterior border. As shown in several other specimens, the exopodite ends in a more or less long spoon-shaped segment bearing on its lower surface a broad groove. No setæ appear to be attached to this, but both anterior and posterior margins bear numerous small, apparently movable spines. From the groove along the ventral side of the remainder of the exopodite arise numerous long slender filaments which become progressively shorter toward the tip. This specimen shows that they are not cylindrical, but are flattened along opposite faces, at least at their distal ends. While no connection can be seen between adjacent setæ, they seem to stay together like the barbs on a feather.
Measurements: Length, 33 mm., width at back of cephalon, 16 mm.; from front of cephalon to back of hypostoma, 6 mm.
Specimen No. 219 (pl. 2, fig. 6; pl. 4, fig. 4).
Illustrated: Amer. Jour. Sci., vol. 13, 1902, pl. 4, fig. 1, pl. 5, fig. 4 (photograph and drawing).
The endopodites of most of the appendages of the thorax are well shown, and occasional portions of exopodites. The coxopodites are long, flattened, and do not taper much. The anterior and posterior edges of the basipodites of the endopodites of the first two segments are approximately parallel, but on the succeeding endopodites the basipodites and ischiopodites are triangular in form, with the apex backward. In successive endopodites toward the posterior end, the angle made by the backward-directed sides of the basipodites becomes increasingly acute, so that in some of the posterior appendages this segment is wider than long. The ischiopodite shows a similar increase of width and angularity on successive segments, and the meropodites and carpopodites also become wider on the posterior segments, and even triangular in outline toward the back of the thorax and on the pygidium.
Along the median portion of the axial lobe the specimen has been cleaned until the inner side of the ventral membrane was reached. Here the test shows on the inner surface at each segment of the thorax a series of low ridges which are roughly parallel to the axial line, but [157] which really converge in an anterior direction. Between the ridges are shallow canoe-shaped depressions, which have the appearance of areas for the insertion of muscles.
Measurements: Length, 31 mm.; width at back of head, 15 mm.; distance, in a straight line, from point of insertion of the right antennule to its tip, 14.25 mm.; it projects 12 mm. beyond the cephalon.
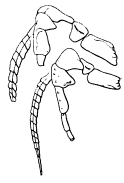
Fig. 43.—Triarthrus becki Green. Drawing to represent the writer's interpretation of the appendages of specimen 218. Drawn by Miss Wood. × 10.
Specimen No. 218 (pl. 6, fig. 3; text fig. 43).
This specimen is a large one, developed from the lower side, but retains only the endopodites of a few appendages. The cephalon and anterior portion of the thorax are missing.
Professor Beecher had a drawing made to show the appendages on the right-hand side of the last two segments of the thorax, seen of course from the ventral side. This drawing shows well the broadening of the basipodite, ischiopodite, and meropodite, while the coxopodite is thick and heavy, and the inner end of the gnathobase somewhat rugose. Almost every segment of the endopodites has one or more pits for insertion of spines, these being along the anterior or posterior margins. The exopodites lack the setæ, but show no unusual features.
Specimen No. 222 (pl. 4, fig. 5).
Illustrated: Amer. Jour. Sci., vol. 47, 1894, pl. 7, fig. 3 (drawing).
A small specimen, developed from the lower side, and used by Professor Beecher to illustrate the form of the segments of the endopodites of the pygidium. In addition to this, it shows very well the form of the endopodites of the thorax. All of the appendages on the specimen are shifted to the left of their normal position. This specimen differs from most of the others in that the segments of the endopodites do not lie with their greatest width in the horizontal plane, but were embedded vertically, with the posterior edge downward. From this circumstance they retain their natural shape, and it is seen that they are naturally flattened, with about the same thickness in proportion to length and width as in some of the modern isopods (Serolis, for instance). In even the most anterior of these endopodites (that of the second segment) the ischiopodite, meropodite, and carpopodite are [158] triangular in shape, with the point backward, but in all the endopodites at the anterior end of the thorax, the triangle has a very obtuse angle at the apex, and the base is much longer than the perpendicular. On the other hand, those of the pygidium, which were figured by Beecher, have a number of short wide segments, all wider than long, and, excepting the dactylopodites, triangular in form.
Measurements: Length, 8.75 mm.; width at back of cephalon, about 4 mm.
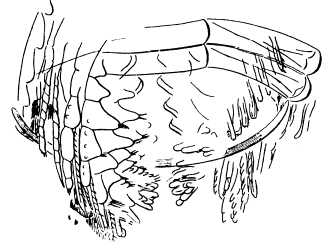
Fig. 44.—Triarthrus becki Green. Appendages of the posterior part of the thorax and pygidium of specimen 230. Inked by Miss Wood from a tracing made under the direction of Professor Beecher.
Specimen No. 230 (pl. 5, fig. 3; text fig. 44).
Illustrated: Amer. Jour. Sci., vol. 47, 1894, pl. 7, fig. 2 (drawing); Ibid., vol. 13, 1902, pl. 2, fig. 2.
An entire specimen of medium size, developed from the ventral side. It seems to have been the first one to yield to Professor Beecher any satisfactory knowledge of the appendages of the pygidium. There are five endopodites, all on one side, which appear to belong here. The segments in this region are characterized by their short, wide, triangular form. At the apex of each is a small tuft of spines or short hairs, and the ventral surfaces of some of the endopodites show pits for the insertion of spines.
Measurements: Length, 21 mm.; width at back of cephalon, 10 mm.
Cryptolithus tessellatus Green.
Specimen No. 233 (pl. 7, fig. 1; text fig. 45).
This is the best preserved entire specimen. It is developed from the lower side, and shows the hypostoma, antennules, and a few fragmentary appendages of the cephalon, the outer portions of the exopodites of thorax and pygidium on both sides, and the endopodites on the left side.
The hypostoma is imperfectly preserved and is turned completely around, so that the anterior margin is directed backward, and the posterior one is so much in the shadow that it does not show well in any of the photographs. The form is, however, essentially like [159] that of Trinucleoides reussi (Barrande), the only other trinucleid of which the hypostoma is known, except that the border does not extend so far forward along the sides, and it is much smaller.
The antennules are not inserted close to the hypostoma, as in Triarthrus, but at some distance from it, and, as nearly as can be determined, directly beneath the antennal pits which are seen near the front of the glabella in many species of trinucleids.
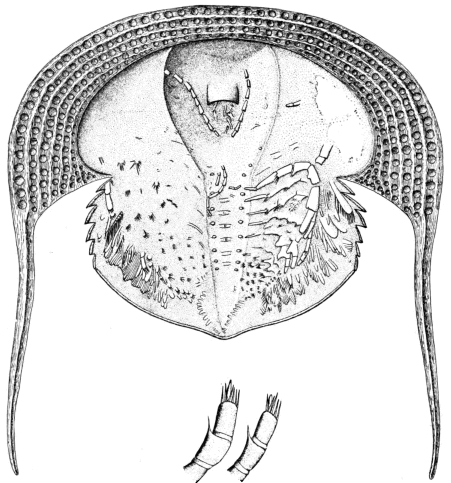
Fig. 45.—Cryptolithus tessellatus Green. Drawing of specimen 233, made by Professor Beecher. × 9. Below are parts of two of the endopodites of specimen 236, showing the interarticular membranes. × 41.
The antennules are long, and are composed of far fewer and longer segments than those of Triarthrus. In this specimen they converge backward, cross each other and at the distal end are more or less intertwined.
As is shown in the drawing and photograph, very little can be learned from this individual about the other appendages of the cephalon. A few fragments of exopodites on either side suggest that these members pointed forward and were much like those in Triarthrus, but nothing conclusive is shown.
The exopodites and endopodites of the left side of the thorax are best preserved. The exopodites are above the endopodites, and only that portion exposed from the ventral side which projects beyond the line at which the endopodites bend backward. The endopodite on the left side of the first thoracic segment is the best preserved. It shows seven segments, the outer ones best. The coxopodite is short and narrow, the basipodite somewhat heavier and longer, while the carpopodite and propodite are the widest and strongest segments. The propodite is triangular and flattened, like the segments on the middle and posterior part of the thorax of Triarthrus. At the inner end of the ischiopodite and meropodite are tufts of spines pointing inward and backward. These are not shown on any of the photographs, but may be seen with the light striking the specimen at the proper angle.
It is not possible to count the exact number of limbs, but one gets the impression that on the left side of this specimen there are twenty-one sets of appendages, six of which of course belong to the thorax. On the thorax and anterior part of the pygidium, successive endopodites show the propodites and dactylopodites becoming progressively more slender and shorter, while the ischiopodites, meropodites and carpopodites become shorter and more triangular, and with increasingly large numbers of short spines on their posterior borders. Back of the fourth endopodite on the pygidium it is not possible to make out the detail, but the appearance is of an endopodite consisting of short broad segments fringed at the back with short spines, the ones at the very posterior end appearing to be exceedingly short and rudimentary.
The exopodites are not so well shown as in some others but the setæ are flattened and blade-shaped, and often bear numerous small spines.
Measurements: Length (lacking most of the fringe), 10.5 mm. Width of thorax, 10.5 mm. Length of hypostome, 1.41 mm., width at front, 1.46 mm. The distance from back of fringe to end of antennules is 5.4 mm. If straightened out, the left antennule would be about 6.1 mm. long. In the first 3.1 mm., there are only ten segments, so that the average length of a segment is 0.31 mm. The distance from the inner end of the endobase of the first segment of the thorax to the outer end of the meropodite is 2.43 mm., and from that point to the end of the dactylopodite 2.47 mm. making the total length 4.90 mm. These measurements are taken from the photograph. Measurements taken from Professor Beecher's drawing, which was made with the camera-lucida, give a total length of 4.57 mm., the distance to the outer end of the meropodite being 2.3 mm. and thence to the tip of the dactylopodite 2.27 mm. Detailed measurements of the segments, on the photograph, are as follows: coxopodite, 0.321 mm.; basipodite, 0.78 mm.; ischiopodite, 0.68 mm.; meropodite, 0.642 mm.; carpopodite, 0.642 mm.; propodite, 1.01 mm., dactylopodite, 0.825 mm.
Specimen No. 235 (pl. 7, fig. 2; pl. 8, fig. 3; pl. 9, figs. 1, 2).
Illustrated: Amer. Jour. Sci., vol. 49, 1895, pl. 3, figs. 5, 6.
Specimens 235 and 236 were originally parts of an entire Cryptolithus, but, as Professor Beecher has explained, the specimen was cut in two longitudinally on the median line, and the halves transversely just back of the cephalon, so that each now represents one half of a thorax and pygidium. Both halves have been cleaned from both upper and lower side, a perfectly marvelous piece of work, for the thickness is no greater than that of a thin sheet of paper, and the soft shale of the matrix has a very slight cohesive power.
Both sides of specimen 235 were figured, but the dorsal side was apparently then somewhat [161] less fully developed than at present. On plate 9 are two figures in which specimens 235 and 236 are brought together again, and both dorsal and ventral sides illustrated.
On the dorsal side, specimen 235 shows portions of three exopodites which lie in a direction roughly parallel to the outer portions of the endopodites on the lower side, that is, their direction if projected would reach the axis in an acute angle back of the end of the pygidium. The setæ stand at right angles to the shaft, and on a portion of it 0.5 mm. long there are seven of them. This is a fragment of an exopodite near the front of the thorax, and the setæ, which are flattened, are about 1.63 mm. long.
On the ventral side this same specimen shows incomplete endopodites and exopodites of about seventeen segments, six of which would belong to the thorax and the remainder to the pygidium. The greater part of the appendages belonging to the pygidium are exceedingly small (about 0.15 mm. long) and so incompletely exposed that the structure can not be definitely made out.
The endopodites of the thoracic segments all lack the greater part of their proximal segments and are all of practically the same form. They turn abruptly backward at the outer end of the meropodite, and the carpopodite of each is greatly widened, projects inward and is armed with tufts of spines. The propodite and dactylopodite are wide, flattened, and taper but slightly outward, the dactylopodite bearing on its distal end a tuft of spines. On several of the endopodites, the meropodites are visible and they bear on their inner ends fringes of spines pointing inward. Behind these well preserved appendages the proximal segments of several endopodites are visible, and a regular succession of flattened, oval bodies armed with numerous forward-pointing spines. These latter bodies Professor Beecher took to be leaf-like exopodites, which they certainly resemble, and as they lie beyond the line of endopodites they probably do belong to the outer halves of the appendages.
The exopodites under the thorax are long, the shaft shows numerous short segments, and is in each case bent backward, though not through a right angle. They extend considerably beyond the endopodites. The setæ do not diverge from the shaft at a right angle as on the dorsal side of this same specimen, but at an acute angle, indicating that they were not rigid. The individual hairs are broad and blade-shaped, frequently with a linear depression along the median line, perhaps due to collapse of the internal tube.
Measurements: The greatest length of the fragment in its present state is 5 mm. The dactylopodite of the second endopodite (without terminal spines) is 0.18 mm. long, the propodite 0.23 mm. long and 0.15 mm. wide; the carpopodite is 0.24 mm. long and 0.38 mm. wide. All measurements were made on the photographs.
Specimen No. 236 (pl. 7, figs. 3-5; pl. 9, figs. 1, 2; text fig. 45).
The right half of the same thorax and pygidium as specimen No. 235.
The specimen is cleaned from both upper and lower sides and, the dorsal test being removed, reveals the long blade-like setæ of the exopodites, each blade being concave along its median line. They are long on the exopodites of the thoracic segments, but become shorter, without, however, any visible change of form on the pygidium. Although the posterior end is not well preserved, one gets no suggestion from a study of this side of the specimens that the exopodites of the posterior end are in any striking way different from those of segments further forward. The tips of some of the setæ show minute spines, one to each blade.
On the ventral side are a number of endopodites, but they are more fragmentary than those of the other half of the specimen. Some of the exopodites are well shown, the blades being in all cases broken from the shaft. Two of the endopodites of this specimen are of especial interest, as they have interarticular membranes between the last three segments. Professor Beecher made a drawing of one of these which he placed under his pen drawing (text fig. 45).
Measurements: The specimen is 5 mm. long from the front of the second thoracic segment to the end of the pygidium. The setæ on the exopodites of the anterior thoracic segments are 1.7 mm. long, as exposed from the dorsal side. Some of those on the posterior part of the pygidium, only incompletely exposed, are 0.31 mm. long.
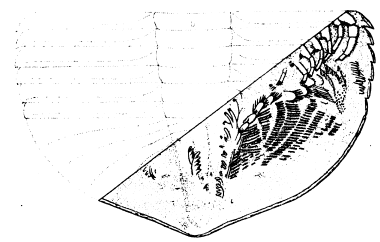
Fig. 46. Cryptolithus tessellatus Green. A part of a thorax and pygidium, showing appendages. Drawn by Professor Beecher. Specimen 238. × 10.
The dactylopodite of the first endopodite showing the articular membranes is 0.23 mm. long and 0.13 mm. wide. The propodite is of the same length and 0.17 mm. wide. The interarticular membrane between them is 0.066 mm. thick. The spines on the dactylopodite of this appendage are 0.15 mm. long. All measurements were made on photographs.
Specimen No. 238 (pl. 8, fig. 4; text fig. 46).
A triangular specimen consisting of the greater part of a pygidium and parts of all the thoracic segments. Under the thorax the specimen has been so cleaned that the outer portions of the endopodites are well shown, while under the pygidium the greater part of the endopodites seem to have been removed, disclosing the setæ of the exopodites. As in other specimens, the endopodites of the thorax turn backward at the distal end of the carpopodite, which is broad and curved, and bears a tuft of spines on the posterior margin. The dactylopodites seem to preserve their natural shape, and are very nearly cylindrical in form. Under the pygidium are several sets of overlapping fringes of setæ of exopodites, and along the edge of the dorsal furrow, a number of fragments of segments of what may be coxopodites while with them are a number of fragmentary shaft of exopodites.
Measurements: The pygidium is 3.3 mm. long, the thorax 3 mm.
BIBLIOGRAPHY.
Agassiz, L.
1873.—Discovery of the basal joint of legs of trilobites. Amer. Nat., vol. 7, pp. 741-742.
Angelina N. P.
1854.—Palæontologia Scandinavica, pars 1, Crustacea formationis transitionis.
Audouin, J. V.
1821.—Recherches sur les rapports naturels qui existent entre les trilobites et les animaux articulés. Ann. Gen. Sci. Phys. Nat. Bruxelles, vol. 8, p. 233, pl. 26. 1822. Isis (Encycl. Zeitung), Oken., vol. 10, p. 87, pl. 1, No. 4, figs. 1-5.
Barrande, J.
1852.—Systême Silurien du centre de la Bohême, vol. 1, pp. 226-230, and 629, pl. 30, figs. 38, 39.
1872.—Ibid., vol. 1, Suppl., p. 180, pl. 4.
Barth, Hermann von.
1875.—Die Stellung der Trilobiten in zoologischen Systeme. Das Ausland, 26. Jahrg., p. 2 5.
Beecher, C. E.
1893.—On the thoracic legs of Triarthrus. Amer. Jour. Sci. (3), vol. 46, pp. 367-370, 467-470, text figs. 1-3.
1894 A.—On the mode of occurrence, and the structure and development of Triarthrus becki. Amer. Geol., vol. 13, pp. 38-43, pl. 3.
1894 B.—The appendages of the pygidium of Triarthrus. Amer. Jour. Sci. (3), vol. 47, pp. 298-300, pl. 7, text fig, 1.
1895 A.—Further observations on the ventral structure of Triarthrus. Amer. Geol., vol. 15, pp. 91-100, pls. 4-5.
1895 B.—Structure and appendages of Trinucleus. Amer. Jour. Sci. (3), vol. 49, pp. 307-311, pl. 3.
1895 C.—The larval stages of trilobites. Amer. Geol., vol. 16, pp. 166-197, pls. 8-10.
1896 A.—The morphology of Triarthrus. Amer. Jour. Sci. (4), vol. 1, pp. 251-256, pl. 8; Geol. Mag., dec. 4, vol. 3, pp. 193-197, pl. 9.
1896 B.—On a supposed discovery of the antennas of trilobites by Linnæus in 1759. Amer. Geol., vol. 17, pp. 303-306, text figs. 1-3.
1897 A.—Outline of a natural classification of trilobites. Amer. Jour. Sci. (4), vol. 3, pp. 89-106, 181-207, pl. 3.
1897 B.—Remarks on Kingsley's "Systematic position of the trilobites." Amer. Geol., vol. 20, pp. 38-40.
1900.—Trilobita. Eastman-Zittel Text-book of Paleontology, vol. 1, pp. 607-638, text figs. 1261-1331; ed. 2, 1913, p. 700. London.
1901.—Structure and development of trilobites. In "Studies in Evolution," pp. 109-225. New York and London.
1902.—The ventral integument of trilobites. Amer. Jour. Sci. (4), vol. 13, pp. 165-174, pls. 2-5, text fig. 1; Geol. Mag., dec. 4, vol. 9, pp. 152-162, pls. 9-11, text figs. 1-8.
Bernard, H. M.
1892.—The Apodidæ.
1893.—Trilobites with antennæ at last! Nature, vol. 48, p. 582.
1894.—The systematic position of the trilobites. Quart. Jour. Geol. Soc., London, vol. 50, pp. 411-434, text figs. 1-17.
1895 A.—The zoological position of the trilobites. Science Prog., vol. 4, pp. 33-49.
1895 B.—Supplementary notes on the systematic position of the trilobites. Quart. Jour. Geol. Soc., London, vol. 51, pp. 352-360, figs. A-C.
Beyrich, E.
1846.—Untersuchungen ueber Trilobiten. 2. Stück, p. 30, pl. 4, fig. 1c.
Billings, E.
1870.—Notes on some specimens of Lower Silurian trilobites. Quart. Jour. Geol. Soc., London, vol. 26, pp. 479-486, pls. 31-32. Abstract in Geol. Mag., vol. 7, p 291, and Nature, vol. 2, p. 94.
Brongniart, A.
1822.—Histoire naturelle des crustacés fossiles. Paris.
Brünnich, F. E.
1781.—Beskrivelse over trilobiten, en dyreslaegt og dens arter, med en ney arts aftegning. Nye Samlig of det Kong. Danske Vidensk. Selskabs. Skriften, Copenhagen.
Burling, L. D.
1916.—Pædeumias and the Mesonacidæ, with description of a new species, having at least 44 segments, from the Lower Cambrian of British Columbia. Ottawa Nat., vol. 30, pp. 53-58, pl. 1.
1917.—Was the lower Cambrian trilobite supreme? Ibid., vol. 31, pp. 77-79, text figs. 1-2.
Burmeister, H.
1843.—Die Organisation der Trilobiten. Berlin.
1846.—The organization of trilobites, deduced from their living affinities. Eng. translation, Ray Society, London.
Calman, W. T.
1909.—Crustacea, in "A treatise on zoology," edited by Sir Ray Lankester. London.
1919. Dr. C. D. Walcott's researches on the appendages of trilobites. Geol. Mag., dec. 6, vol. 6, pp. 359-363, pl. 8, text fig. 1.
Carpenter, G. H.
1903.—On the relationships between the classes of Arthropoda. Proc. Roy. Irish Acad., vol. 24, pp. 320-360, pl. 6.
Castelnau, F. DE.
1843.—Systeme Silurien de l'Amérique Septentrionale, p. 15, pl. 2, figs. 1, 4.
Clarke, J. M.
1888.—The structure and development of the visual area in the trilobite, Phacops rana Green. Jour. Morph., vol. 2, pp. 253-270, pl. 1.
Crampton, G. C.
1916.—The phylogenetic origin and the nature of the wings of insects, according to the paranotal theory. Jour. New York Entomol. Soc., vol. 24, pp. 1-39, pls. 1, 2.
1919.—The evolution of the arthropods and their relatives, with especial reference to insects. Amer. Nat, vol. 53, pp. 143-179.
Dalman, J. W.
1826.—Om Palæaderna eller de så kallade Trilobiterna. Stockholm, Acad. Handl., pp. 113-152, 226-294.
1828.—Ueber die Palæaden, oder die sogennanten Trilobiten. Nuremberg.
Dana, J. D.
1871.—On the supposed legs of the trilobite, Asaphus platycephalus. Amer. Jour. Sci. (3), vol. 1, pp. 320-321, 386; Ibid. (3), vol. 3, 1872, pp. 221-222. Also printed in Ann. Mag. Nat. Hist, vol. 7, 1871, pp. 366, 451.
Dekay, J. E.
1824.—Observations on the structure of trilobites, and description of an apparently new genus. Ann. Lye. Nat. Hist. New York, vol. I, p. 174, 2 pls.; Isis (Encycl. Zeit.), Oken, 1825 and 1832.
Dollo, L.
1910.—La paléontologie éthologique. Bull. Soc. Beige de Geol., Pal., et d'Hydrol., vol. 23, pp. 377-421, figs. 1-13, pls. 7-11.
Eichwald, E. VON.
1825.—Geognostico-zoologicæ per Ingriam Marisque Baltici Provincias nee non de trilobitis observationes. Section 45.
1858.—Beiträge zur geographischen Verbreitung der fossilen Thiere Russlands. Bull. Soc. Imp. des Natural, de Moscou, vol. 30, 1855-1857, p. 204.
1860.—Lethæa Rossica, pl. 21.
1863.—Beiträge zur nähern Kenntniss der in meiner Lethæa Rossica beschriebenen Ilænen. Bull. Soc. Imp. des Natural, de Moscou, vol. 36, p. 408.
Emmrich, H. F.
1839.—De trilobitis dissertatio petrefactologica, etc. Berlin.
Exner, S.
1891.—Die Physiologic der facettirten Augen von Krebsen und Insecten. Leipzig and Vienna. Pp. 33-35, pl. 2, figs. 18-19.
Finch, G. E.
1904.—Notes on the position of the individuals in a group of Nileus vigilans found at Elgin, Iowa. Proc. Iowa Acad. Sci. for 1903, vol. 11, pp. 179-181, pl. 14.
Gegenbaur, C.
1878.—Elements of comparative anatomy. Eng. ed. (Bell and Lankester). London.
Goldfuss, A.
1828.—Observation sur le place qu'occupent les trilobites dans le règne animal. Ann. Sci. Nat., Zoologie, vol. 15, p. 83, pl. 2, figs. 5, 7, 9, 10.
Green, J.
1839 A.—The inferior surface of the trilobite discovered. The Friend, Philadelphia, March 16.
1839 B.—The inferior surface of the trilobite discovered. Illustrated, with colored models. Philadelphia.
1839 C.—Remarks on the trilobites. Amer. Jour. Sci. (1), vol. 37, p. 25 et seq.
1840.—An additional fact, illustrating the inferior surface of Calymene bufo. Ibid., vol. 38, p. 410.
Handlirsch, A.
1906.—Ueber Phylogenie der Arthropoden. Verhandl. d. k. k. zool.-bot. Gesell., Vienna, Jahrg. 1906, pp. 88-103.
1907.—Functionswechsel einiger Organe bei Arthropoden. Ibid., Jahrg. 1907, pp. 153-158.
1908.—Die fossilen Insekten. Leipzig.
1914.—Eine interessante Crustaceenform aus der Trias der Vogesen. Verhandl. d. k. k. zool.-bot. Gesell., Vienna, Jahrg. 1914, pp. 1-7, pls. 1, 2.
Hawle, I., and Corda, A. J. C.
1847.—Prodrom einer Monographie der boehmischen Trilobiten, pp. 9, 24, 56, pl. 2, fig. 10; pl. 3, fig. 15; pl. 4, fig. 33b-g.
Jaekel, O.
1901.—Beiträge zur Beurtheilung der Trilobiten, Theil I. Zeits. d. deutsch. geol. Gesell., Bd. 53, pp. 133-171. Pis. 4-6, text figs. 1-30.
Kingsley, J. S.
1897.—The systematic position of the trilobites. Amer. Geol., vol. 20, pp. 33-38.
Koenen, A. von.
1872.—Ueber die Organisation der Trilobiten. Verhandl. d. naturhist. Ver. d. preuss. Rheinl. u. Westphalen, vol. 29, C, pp. 93-95.
1880.—Ueber die Unterseite der Trilobiten. Neues Jahrb. f. Min.., Geol., u. Pal,, Bd. 1, pp. 430-432. pl. 8.
Lang, A.
1891.—Text-book of comparative anatomy, Eng. ed. (Bernard). London.
Lankester, E. R.
1881.—Observations and reflections on the appendages and on the nervous system of Apus cancriformis. Quart. Jour. Micros. Soc., vol. 21, pp. 343-376.
Laurie, M.
1911.—A reconstructed trilobite. Nature, vol. 88, p. 26.
Lindstroem, G.
1901.—Researches on the visual organs of the trilobites. K. svenska Vet.-Akad. Handl., new ser., vol. 34, pp. 1-86, pls. 1-6.
Linné, K.
1759.—Petrificatet entomolithus paradoxus sådant, som det finnes uti Hans Excellence Riks. Rådets Högoälborne Herr Grefve C. G. Tessins Samling. K. svenska Vet.-Akad. Handl., vol. 20, pp. 21, 22, pl. 1, fig. 1.
Matthew, W. D.
1893.—On antennæ and other appendages of Triarthrus becki. Amer. Jour. Sci. (3), vol. 46, pp. 121-125, pl. 1; Trans. New York Acad. Sci., vol. 12, pp. 237-241, pl. a.
McCoy, F.
1846.—A synopsis of the Silurian fossils of Ireland, p. 42.
Mickleborough, J.
1883.—Locomotory appendages of trilobites. Jour. Cincinnati Soc. Nat. Hist., vol. 6, pp. [167] 200-204; Geol. Mag., dec. 3, vol. 1, 1884, pp. 80-84; Amer. Jour. Sci. (3), vol. 27, 1884, p. 409. Reviewed by Dames, Neues Jahrb. f. Min., Geol., u. Pal., Bd. 1, 1885, p. 477.
Miller, S. A.
1880.—Silurian ichnolites, with definitions of new genera and species. Jour. Cincinnati Soc. Nat. Hist, vol. 2, pp. 217-218, fig.
Milne-Edwards, H.
1881.—Compte rendu des nouvelles recherches de M. Walcott relatives à la structure des trilobites, suivi de quelques considérations sur l'interprétation des faits ainsi constatés. Ann. Sci. Nat, Zoologie, ser. 6, vol. 12, pp. 1-33, pls. 10-12. Paris.
Moberg, J. C.
1902.—Bidrag till Kännedomen om trilobiternas byggnad. Geol. Fören Förhandl., Bd. 24, pp. 295-302; pl. 3, text fig. 1.
1907.—Om ett gätfultt fossil frän sveriges olenidskiffer samt en kort ofversigt af viktigase data rorande trilobiternas ventrala skelettdelar. Ibid., Bd. 29, Heft 5, pp. 265-272, pl. 4, fig. 2; pl. 5, fig. 1.
Œhlert, D. P.
1896.—Résumé des derniers travaux sur l'organisation et le developpement des trilobites. Bull. Soc. Géol. France, ser. 3, vol. 24, pp. 97-116, text figs. 1-34.
Packard, A. H.
1872.—On the development of Limulus polyphemus. Mem. Boston Soc. Nat. Hist., vol. 2, pp. 155-202, pls. 3-5.
1880.—The structure of the eye of trilobites. Amer. Nat., vol. 14, pp. 503-508.
1882.—On the homologies of the crustacean limb. Ibid., vol. 16, pp. 785-799, figs. 11, 12.
Pander, C.
1830.—Beiträge zur Geognosie des russischen Reiches. St. Petersburg.
Peach, B. N.
1882.—On some fossil myriopods from the Lower Old Red Sandstone of Forfarshire. Proc. Roy. Physical Soc., Edinburgh, vol. 7, pp. 177-187, pl. 2.
1899.—O some new myriopods from the Palæozoic rocks of Scotland. Ibid., vol. 14, pp. 113-126, pl. 4.
Quenstedt, A.
1837.—Beitrag zur Kenntniss der Trilobiten, mit besonderer Rücksicht auf ihre bestimmte Gliederzahl. Archiv f. Naturg., Berlin, 3. Jahrg., 1 Bd., pp. 337-352.
Raymond, P. E.
1910.—On two new trilobites from the Chazy near Ottawa, Ontario. Ottawa Nat., vol. 24, pp. 129-134, pl. 2.
1917.—Beecher's classification of trilobites, after twenty years. Amer. Jour. Sci. (4), vol. 43, pp. 196-210, text figs. 1-3.
Raymond, P. E., and Barton, D. C.
1913.—A revision of the American species of Ceraurus. Bull. Mus. Comp. Zool., vol. 54, pp. 525-543. pls. 1, 2, 3 text figs. 1-3.
Reed, F. R. C.
1916.—The genus Trinucleus. Pt. 4. Geol. Mag., dec. 6, vol. 3, pp. 121, 122.
Richter, R.
1848.—Bitrag zur Palæeontologie des Thüringer Waldes. Dresden and Leipzig.
Ringueberg, E. N. S.
1886.—A trilobite track illustrating one mode of progression of the trilobites. Proc. Amer. Assoc. Adv. Sci., vol. 35, p. 228 (abstract only).
Ruedemann, R.
1916.—The presence of a median eye in trilobites. Bull. New York State Mus., No. 189. pp. 127-143, pls. 34-36.
Schlotheim, E. F. von.
1823.—Nachträge zur Petrefactenkunde, II. Gotha.
Six, Achille.
1884.—Les appendices des trilobites d'après M. Ch. D. Walcott. Ann. Soc. Geol. du Nord, vol. 11, pp. 228-236.
Spencer, W. K.
1903.—The hypostomic eyes of trilobites. Geol. Mag., dec. 4, vol. 10, pp. 489-492.
Staff, Hans v., and Reck, Hans.
1911.—Ueber die Lebensweise der Trilobiten. Eine entwicklungsmechanische Studie. Gesell. naturforsch. Freunde, Sitzb., pp. 130-146, figs. 1-20.
Sternberg, K. M.
1830.—Ueber die Gliederung und die Füsse der Trilobiten. Isis (Encycl. Zeitung), Oken, p. 516, pl. 5, figs. 1-3.
Stokes, C.
1823.—On a trilobite from Lake Huron. Trans. Geol. Soc., London, ser. 2, vol. 1, p. 208, pl. 27.
Swinnerton, H. H.
1919.—The facial suture of the trilobite. Geol. Mag., dec. 6, vol. 6, pp. 103-110.
Törnquist, S. L.
1896 A.—On the appendages of trilobites. Ibid., dec. 4, vol. 3, p. 142.
1896 B.—Linnæus on the appendages of trilobites. Ibid., pp. 567-569.
Tothill, J. D.
1916.—The ancestry of insects, with particular reference to chilopods and trilobites. Amer. Jour. Sci. (4), vol. 42, pp. 373-383. text figs. 1-8.
Troedsson, G. T.
1918.—Om skanes Brachiopodskiffer. Lunds Universitets Arsskrift, n. f., Avd. 2, Bd. 15, Nr. 3. pp. 57-67, pl. 1, figs. 19-24.
Valiant, W. S.
1901.—Appendaged trilobites. The Mineral Collector, vol. 8, No. 7, pp. 105-112.
Volborth, A. von.
1858.—Ueber die Bewegungs-Organe der Trilobiten. Verhandl. russ. k. mineral. Gesell. zu St Petersburg, 1857-1858, p. 168.
1863.—Ueber die mit glatten Rumpfgliedern versehenen russischen Trilobiten, nebst einem [169] Anhange ueber die Bewegungs-organe und ueber das Herz derselben. Mem. Acad. Imp. Sci. St. Petersburg, ser. 7, vol. 6, No. 2, pp. 44-47, pl. 1, fig. 12.
1866.—Ueber Herrn von Eichwald's Beitrag zu näheren Kenntniss der Illænen. Bull. Soc. Imp. des Natural, de Moscou, vol. 39, p. 40.
Wahlenberg, G.
1821.—Petrificata telluris Suecana examinata a Georgio Wahlenberg. Nova Acta Reg. Soc. Scient. Upsala, vol. 8.
Walcott, C. D.
1875.—Description of the interior surface of the dorsal shell of Ceraurus pleurexanthemus Green. Ann. Lye. Nat. Hist. New York, vol. II, pp. 159-162, pl. 11.
1876.—Preliminary notice of the discovery of the natatory and branchial appendages of trilobites. 28th Rept. New York State Mus. Nat. Hist., adv. sheets, pp. 89-92; published as full report in 1879.
1877.—Notes on some sections of trilobites. 31st Rept. New York State Mus. Nat. Hist., adv. sheets, pp. 61-63, pl. 1; published as full report in 1879. Reviewed by Dames, Neues Jahrb. f. Min., Geol., u. Pal., Bd. 1, 1880, p. 428.
1879.—Notes upon the legs of trilobites. 31st Rept. New York State Mus. Nat. Hist., adv. sheets, p. 64.
1881.—The trilobite: New and old evidence relating to its organization. Bull. Mus. Comp. Zool., vol. 8, pp. 192-224, pls. 1-6.
1884.—The appendages of the trilobite. Science, vol. 3, pp. 276-279, figs. 1-3. Reviewed by Dames, Neues Jahrb. f. Min., Geol., u. Pal.., Bd. 1, 1885, Referate, p. 102.
1894.—Note of some appendages of the trilobites. Proc. Biol. Soc. Washington, vol. 9, pp. 89-97, pl. 1; Geol. Mag., dec. 4, vol. 1, pp. 246-251, pl. 8.
1911.—Middle Cambrian Merostomata. Smithson. Misc. Coll., vol. 57, No. 2, pp. 17-40, pls. 2-7.
1912 A.—Middle Cambrian Branchiopoda, Malacostraca, Trilobita, and Merostomata. Ibid., No. 6, pp. 145-228, pls. 24-34, text figs. 8-10.
1912 B.—New York Potsdam-Hoyt fauna. Ibid., No. 9, pp. 251-304, pls. 37-49.
1913.—Eastman-Zittel Text-book of Paleontology, ed. 2, vol. 1, figs. 1343, 1376, 1377.
1916.—Ann Rept., Secretary Smithsonian Inst, for 1915, pl. 9.
1918.—Appendages of trilobites. Smithsonian Misc. Coll., vol. 67, No. 4, pp. 115-226, pls. 14-42.
Watase, S.
1890.—On the morphology of the compound eyes of arthropods. Johns Hopkins Univ., Studies from Biol. Lab., vol. 4, no. 6, p. 290 (footnote).
Woodward, H.
1870.—Note on the palpus and other appendages of Asaphus, from the Trenton limestone, in the British Museum. Quart. Jour. Geol. Soc., London, vol. 26, pp. 486-488, fig. 1. Abstract in Geol. Mag., dec. 1, vol. 7, p. 292, also in Nature, vol. 2, p. 94.
1871.—On the structure of trilobites. Geol. Mag., dec. 1, vol. 8, pp. 289-294, pl. 8.
1884.—Notes on the appendages of trilobites. Geol. Mag., dec. 3, vol. 1, pp. 162-165, 2 text figs.
1895.—Some points in the life history of the Crustacea in early Palæozoic times. Quart. Jour. Geol. Soc., London, vol. 51, pp. lxx-lxxxviii, 1 pl.
PLATE 1.
Photographs of Triarthrus becki, made by C. E. Beecher.
Fig. 1. Specimen 213. The dorsal test has been removed from the glabella, revealing the outline of the posterior end of the hypostoma, the proximal ends of the antennules, the gnathites, and incomplete endopodites of some appendages, × 5.43.
Fig. 2. Specimen 214. The head of a complete large specimen. Part of the thorax is shown on pl. 3, fig. 6. Note especially the form of the segments of the endopodites and of the anterior coxopodite on the right side, × 7.33.
Fig. 3. Specimen 217. This specimen shows better than any other the form of the gnathites of the cephalon. Note also the setæ of the exopodites under the cheek at the right. The appearance of a hook on the posterior gnathite on the right may be accidental, but it does not show broken edges, × 6.85.
Fig. 4. Specimen 215. The ventral side of the cephalon of a small entire specimen. Shows well the form of some of the gnathites and a few of the endopodites. Note the unusual position of the antennules. × 7.63.
Fig. 5. Specimen 226. This specimen did not photograph well, but is important as showing the exopodites and endopodites emerging from under the cephalon. × about 6.
PLATE I.
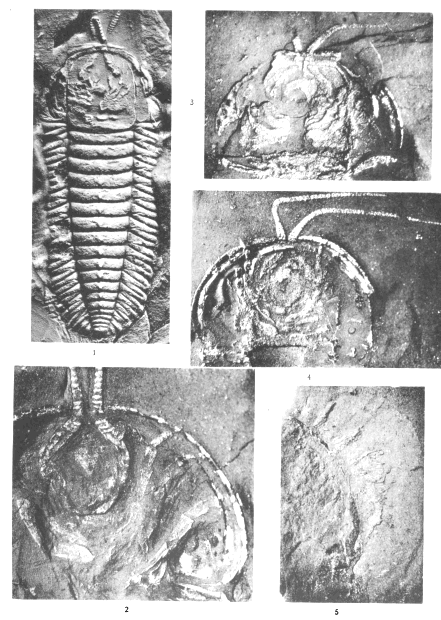
HELIOTYPE CO. BOSTON
PLATE 2.
Photographs of Triarthrus becki, made by C. E. Beecher.
Fig. 1. Specimen 201. The entire specimen, details of which are shown in pl. 3, fig. 4 and pl. 4, figs. 1, 2. The dorsal test has been removed from the anterior segments on the right side. × 4.12.
Fig. 2. Specimen 206. A small individual with the endopodites, and the exopodites minus their setæ; well preserved on the left side. Note the position of the antennules. The course of the facial suture is unusually well shown. × 10.
Fig. 3. Specimen 210. The specimen which served as the main basis for Professor Beecher's first figure of the appendages of the thorax, specimen 206 (fig. 2, this plate) having supplemented it. Note the "normal" position of the antennules and the extension of the appendages from beneath the pleural lobe. Specimens with the antennules in this position may possibly be males. × 4.
Fig. 4. Specimen 205. A small specimen with some of the appendages preserved, especially toward the posterior end, but particularly valuable for the unusually well preserved metastoma. × 11.
Fig. 5. Specimen 211. A small cephalon, cleaned from the ventral side, and showing well the gnathites which approach each other unusually closely on the median line. × 10.5.
Fig. 6. Specimen 219. An entire specimen of medium size, developed from the ventral side. It shows particularly well the "normal" curvature of the antennules, the change in form of the segments of the endopodites from cephalon to pygidium, and, along the axial lobe, the apodemes of the ventral integument. See also pl. 4, fig. 4. × 3.6.
PLATE II.
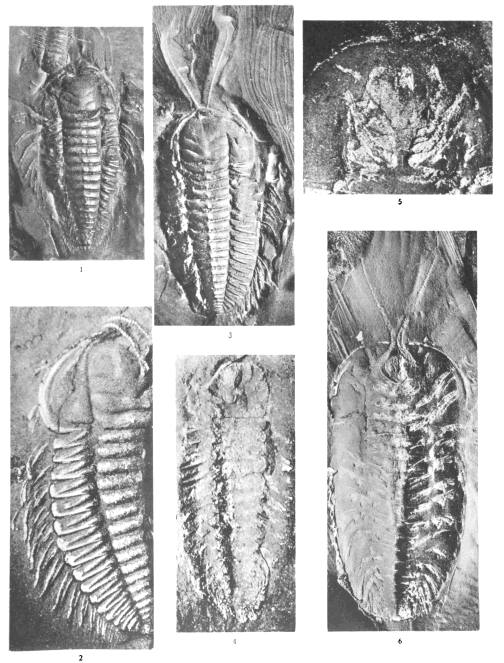
HELIOTYPE CO. BOSTON
PLATE 3.
Photographs of Triarthrus becki, made by C. E. Beecher.
Fig. 1. Specimen 204. See also text fig. 42 and pl. 4, fig. 6. The exopodites and endopodites of the first few segments of this specimen are better preserved than those of any other revealing them from the dorsal side, × 9.5.
Fig. 2. Specimen 220. A large individual exposed from the lower side. It shows well the endopodites and part of the exopodites, and, rather better than any other specimen, the endobases of the coxopodites. × 2.4.
Fig. 3. Specimen 216. A small entire specimen showing considerable of the detail of the appendages of the cephalon, and some of those of the remainder of the body, × 7.4.
Fig. 4. Specimen 201. This figure shows the details of the appendages of the left side and of the pygidium. Note the plate on the median line back of the pygidium, the sockets for spines, and the terminal spines on the anterior endopodites. See also pl. 2, fig. 1 and pl. 4, figs. 1, 2. × 7.1.
Fig. 5. Specimen 207. One half of the posterior part of the thorax and pygidium, showing exopodites and endopodites as seen from the dorsal side, × 7.6.
Fig. 6. Specimen 214. The exopodites have been turned back nearly parallel to the axis of the shell. Notice particularly the long flattened setæ and the spinose spatula-shaped terminal portion of each shaft. See also pl. 1, fig. 2. × 7.
PLATE III.
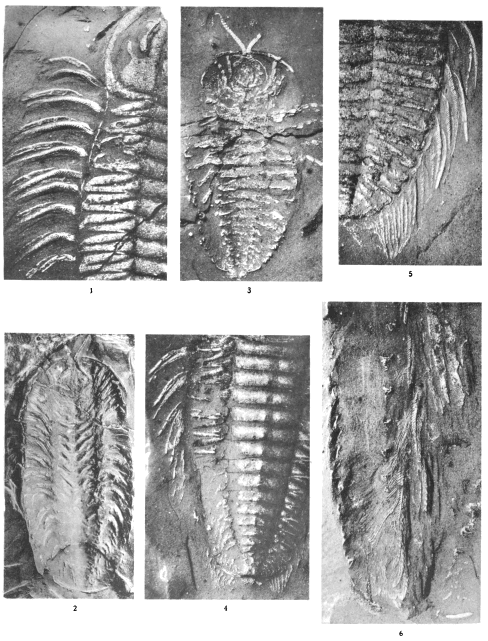
HELIOTYPE CO. BOSTON
PLATE 4.
Photographs of Triarthrus becki, made by C. E. Beecher.
Fig. 1. Specimen 201. Another photograph, similar to fig. 4, pl. 3, but showing more clearly some details of spines on the endopodites. × 12.66.
Fig. 2. Specimen 201. Three appendages on the right side of the thorax. See also pl. 2, fig. 1 and pl. 3, fig. 4. × 12.66.
Fig. 3. Specimen 223. A small crushed specimen which nevertheless shows well the appendages of the right side of the thorax, developed from the ventral side. Note coxopodites, exopodites, and endopodites, and that all appendages are moved equally laterally from their original position. × 11.4.
Fig. 4. Specimen 219. Another photograph, with different lighting, of the individual shown in pl. 2, fig. 6. This print brings out better the coxopodites and the folds of the ventral membrane. × 3.23.
Fig. 5. Specimen 222. This specimen is interesting, because it shows the endopodites in what is probably their natural position, that is, in a plane nearly vertical to the plane of the body, instead of being flattened down, as is usually the case. The appendages under the pygidium are unusually well preserved. × 12.
Fig. 6. Specimen 204. Photograph of the entire specimen of which a part is shown in text fig. 42 and pl. 3, fig. 1. × 4.5.
PLATE IV.
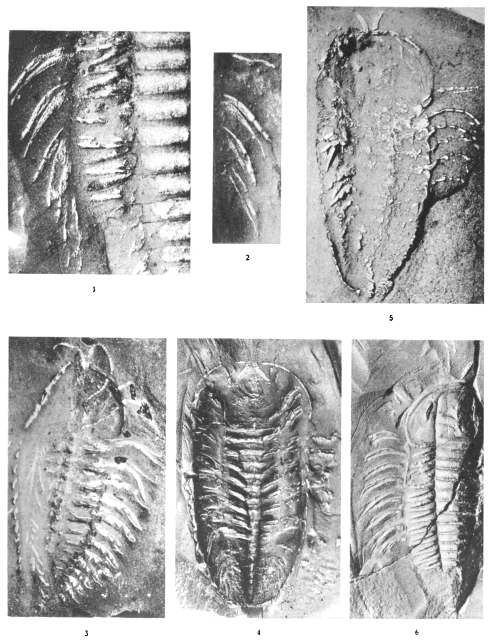
HELIOTYPE CO. BOSTON
PLATE 5.
Photographs of Triarthrus becki, made by C. E. Beecher.
Fig. 1. Specimen 209. Photograph of the pygidium shown in pl. 6, fig. 2. This specimen shows especially well the way in which the exopodites of the pygidium decrease in length backward, × 11.5.
Fig. 2. Specimen 229. The under side of the posterior end of a medium-sized specimen, showing the appendages, especially the endopodites. On and among the limbs are scattered numerous minute spheres of pyrite, of the kind usually known as "trilobite eggs." They do not show very well in the photograph, but can be made out much more clearly with a hand lens, × 12.
Fig. 3. Specimen 230. A specimen showing the appendages of the posterior part of the thorax and the pygidium. The same individual is also shown in text fig. 44. Note particularly the form of the segments of the endopodites, and the spines on them, × 13.
Fig. 4. Specimen 227. The small doubly curved bodies shown in this figure lie under the axial portion of the cephalon and anterior part of the thorax. The specimen still has a very thin coating of matrix between it and the shell. Whether the curved bodies have anything to do with the trilobite is not known, × about 12.
Fig. 5. Specimen 221. A small individual which shows well the exopodites of the posterior part of the thorax. Note the spatulate terminations and the spines of the shaft, × 11.
Fig. 6. Specimen 202. Posterior part of the thorax and pygidium, showing endopodites and exopodites projecting under the dorsal test. Note the spiniferous plate on the median line, and the large opening in the anterior portion of it. × 9.75
PLATE V.
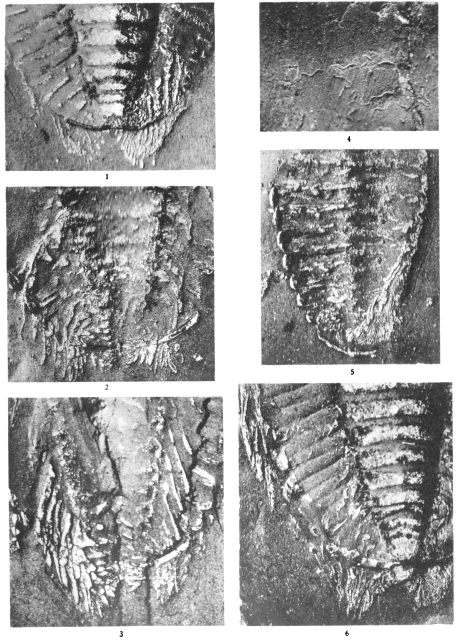
HELIOTYPE CO. BOSTON
PLATE 6.
All figures except 4 and 5, from photographs by C. E. Beecher.
Fig. 1. Triarthrus becki. Specimen 203. A well preserved small individual, showing the appendages of the right side of the thorax. × 11.46.
Fig. 2. Triarthrus becki. Specimen 209. A well preserved individual, showing the antennules and some appendages of thorax and pygidium. For detail of the pygidium, see pl. 5, fig. 1. × 4.
Fig. 3. Triarthrus becki. Specimen 218. Ventral side of the pygidium and greater part of the thorax of an individual of medium size. Note especially the relation of exopodites to endopodites of the last two thoracic segments. A drawing of these appendages is shown on text fig. 43. × 4,3.
Figs. 4 and 5. Endopodites, probably from a species of Calymene. These specimens, with several others, are on a small slab of limestone from the Point Pleasant (Trenton) beds opposite Cincinnati, Ohio. Specimen in the U. S. National Museum. Photographs by R. S. Bassler.
Fig. 6. Acidaspis trentonensis Walcott. Both the specimen, No. 245, and the photograph are poor, but show that in this genus the endopodites are like those of Triarthrus. × 8.5.
Fig. 7. Cryptolithus tessellatus Green. Specimen 234. This specimen shows well the backward directed antennules and also the outer segments of some of the cephalic endopodites. × 11.
PLATE VI.
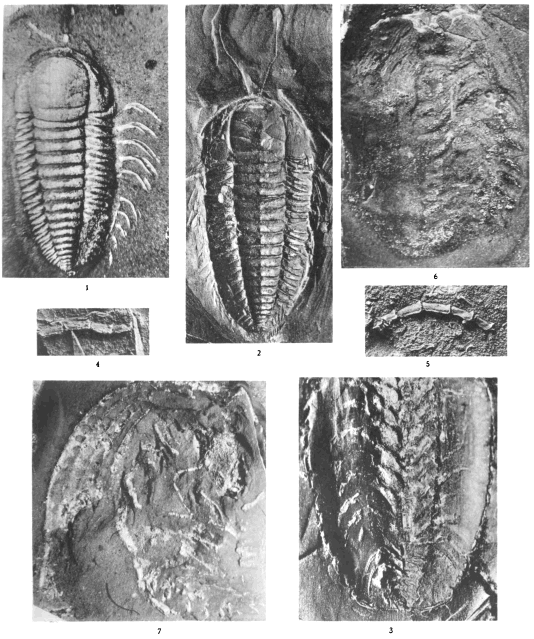
HELIOTYPE CO. BOSTON
PLATE 7.
Photographs of Cryptolithus tessellatus Green, made by C. E. Beecher.
Fig. 1. Specimen 233. The best preserved individual, the one from which Professor Beecher's drawing (text fig. 45) was made, and which served as the principal basis for the restoration (text fig. 20). Note the long, backward directed antennules, the abrupt backward turn of the outer portions of the endopodites, the way in which the exopodites extend beyond the endopodites, and the fact that alt are beneath the cover of the dorsal shield. The hypostoma is turned entirely around. × 10.9.
Fig. 2. Specimen 235. Half of the thorax and pygidium, with the appendages revealed from the ventral side. Note the abrupt manner in which the outer portions of the endopodites are turned backward. See also pl. 8, fig. 3, and pl. 9, fig. 1 (right half). × 14.45.
Fig. 3. Specimen 236. Detail from fig. 4, to show the blade-like setæ of the exopodites and the numerous terminal spines of the endopodites. × 30.
Fig. 4. Specimen 236. The appendages of the thorax and pygidium, seen from the lower side. Specimen 236 is the right half of the same individual from which specimen 235 was obtained. Note the interarticular membranes between the segments of the endopodites and the blade-like setæ of the exopodites. See also pl. 9, fig. 1 (left side). × 19.
Fig. 5. Specimen 236. The same specimen, seen from the dorsal side, showing, when the test is removed, the long blade-like setæ of the exopodites. See also pl. 9, fig. 2 (right half). × 19.
PLATE VII.
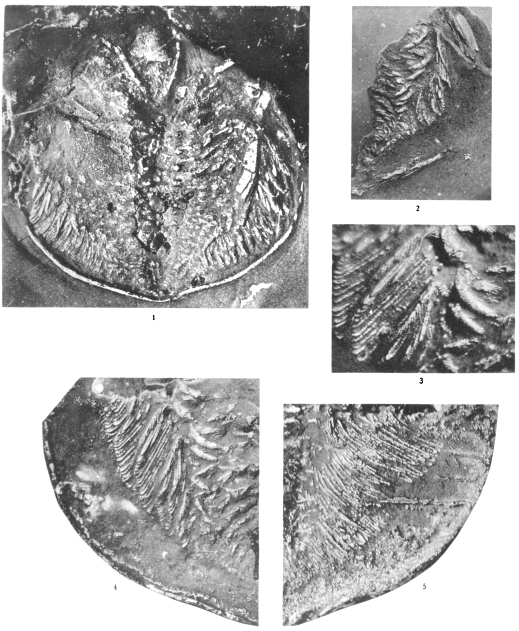
HELIOTYPE CO. BOSTON
PLATE 8.
Photographs of Cryptolithus tessellatus Green, made by C. E. Beecher.
Fig. 1. Specimen 231. A nearly complete individual, cleaned from the ventral side and showing obscurely the hypostoma and fragments of numerous appendages. Note the lines of appendifers along the sides of the axial lobe. × 11.
Fig. 2. Specimen 232. Although this is not very well preserved, it shows more of the cephalic appendages than any other. Even so, only just enough is shown to indicate that they were similar to those on the thorax. × 12.
Fig. 3. Specimen 235. Dorsal side of the appendages of the thorax and pygidium. See pl. 7, fig. 2 for the ventral view. On pl. 9, fig. 2 (left side) is a drawing taken from the same specimen. × 11.
Fig. 4. Specimen 238. Part of a thorax and pygidium, seen from the ventral side. The series of heavy segments shown in the upper part do not belong to one appendage, but are the distal ends of several endopodites. See also text fig. 46 for a drawing of this specimen. × 18.
Fig. 5. Specimen 237. Pygidium and part of the thorax, with some of the appendages. × 11.
PLATE VIII.
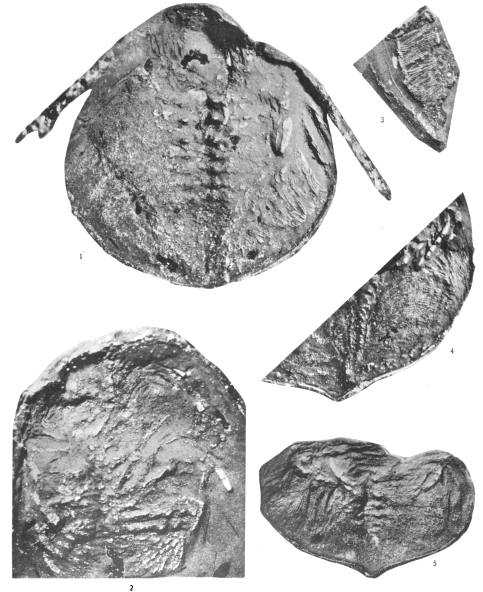
HELIOTYPE CO. BOSTON
PLATE 9.
Cryptolithus tessellatus Green. Upper drawing by C. E. Beecher; lower drawing by Miss F. E. Isham, under the direction of C. E. Beecher.
Fig. 1. Appendages of the thorax and pygidium, seen from the ventral side. These are not restorations, but drawings from the halved individual numbered 236 (right side of drawing) and 235. For photographs of these specimens, see pl. 7, figs. 2, 4. × 20.
Fig. 2. Appendages of the thorax and pygidium, seen from the dorsal side. Same specimen as in fig. 1. For photographs, see pl. 7, fig. 5, and pl. 8, fig. 3. × 20.
PLATE IX.
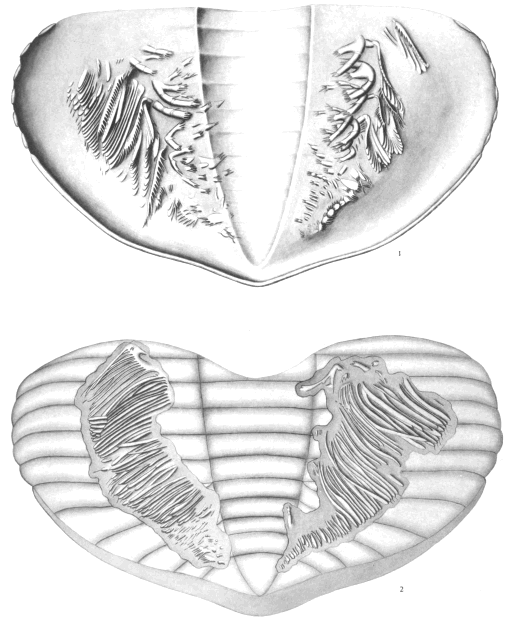
HELIOTYPE CO. BOSTON
PLATE 10.
From photographs made by C. E. Beecher.
Fig. 1. Isotelus latus Raymond. Ventral surface of the specimen in the Victoria Memorial Museum at Ottawa, Canada. Note the large, club-shaped coxopodites and the more slender endopodites. The first large coxopodite back of the hypostoma belongs to the last pair of cephalic appendages. The coxopodite of the appendage in front of it is seen turning in beneath the tip of the hypostoma. × 2.
Fig. 2. Isotelus maximus Locke. The ventral side of the specimen described by Mickleborough and now in the U. S. National Museum. The tips of the hypostoma may be seen at the front, and the first two pairs of coxopodites behind them belong to the last two pairs of appendages of the cephalon. Note how much stronger the coxopodites are than the endopodites. The appendages of the pygidium show but poorly, × 1.45.
PLATE X.
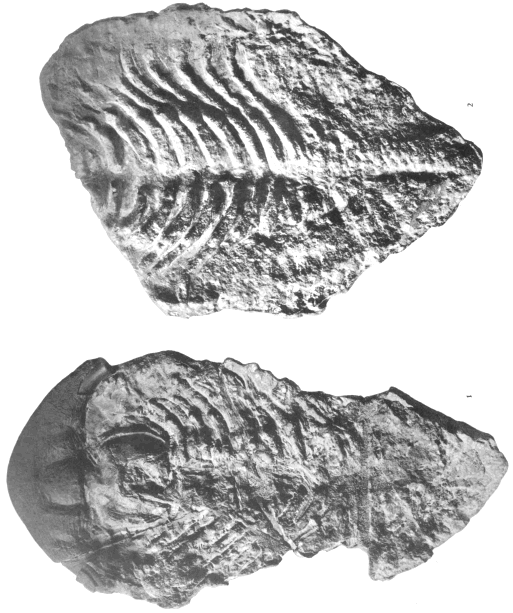
HELIOTYPE CO. BOSTON
PLATE 11.
Ceraurus pleurexanthemus Green. A restoration of the ventral surface and appendages, made by Doctor Elvira Wood, under the supervision of the writer, from data obtained from the translucent slices prepared and described by Doctor Walcott. × 5.
PLATE XI.
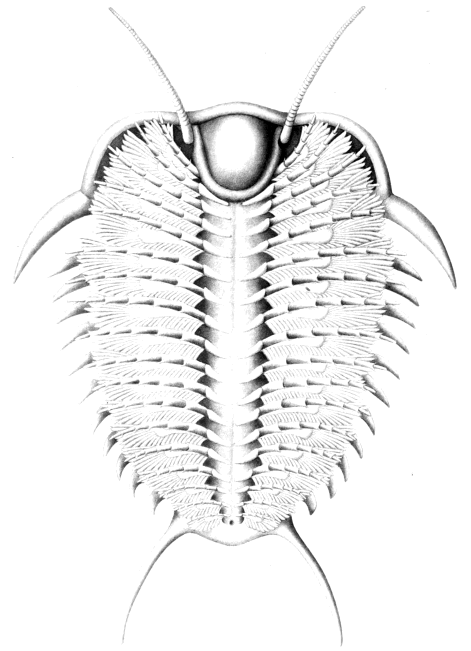
HELIOTYPE CO. BOSTON